Contents
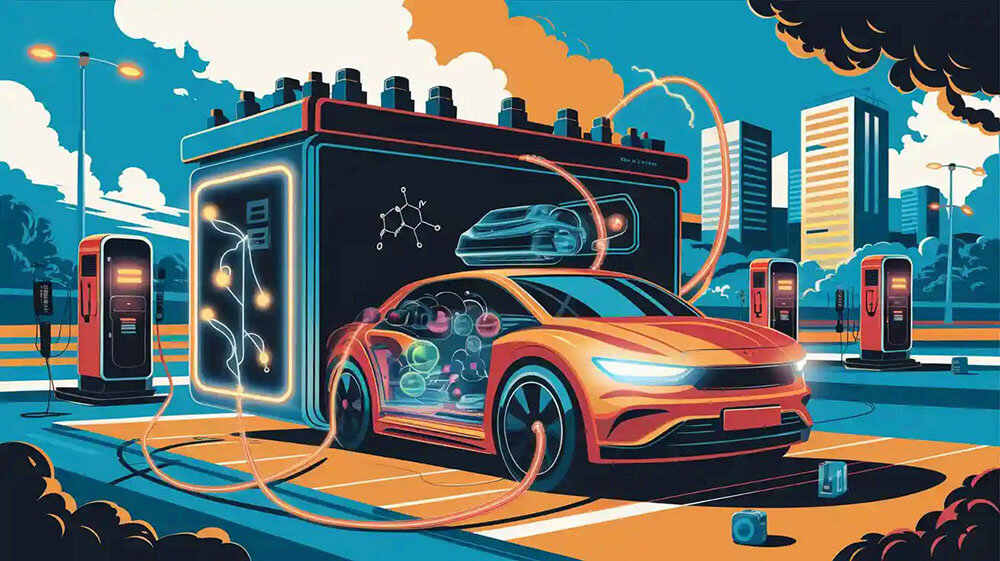
The chemistry of lithium-ion batteries significantly influences the performance and cost of battery electric vehicles. Understanding this chemistry helps you make informed decisions, especially if you operate in fields like transportation, robotics, or infrastructure. It also plays a vital role in the sustainability of electric vehicles lithium battery solutions, ensuring they meet modern energy demands.
Key Takeaways
Learning about lithium-ion batteries helps make electric cars better.
Various battery types, like LCO, LiFePO4, LMO, and NMC, have special benefits in energy, safety, and how long they last.
Using better lithium technology can solve problems with cost, safety, and the environment in electric cars.
Part 1: Overview of Lithium-ion Battery Chemistry
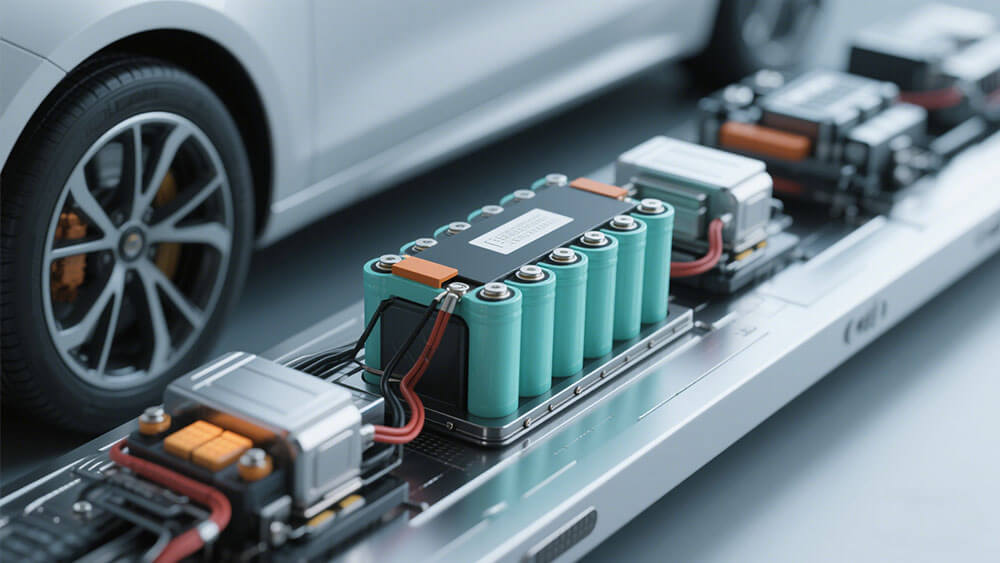
1.1 Key Components of Lithium-ion Batteries
Lithium-ion batteries consist of several critical components that determine their efficiency and safety. The anode and cathode serve as the primary electrodes, facilitating the movement of lithium ions during charge and discharge cycles. The electrolyte acts as a medium for ion transport, while the separator prevents direct contact between the electrodes, ensuring safety. Passive components like connectors and casings maintain the structural integrity of the battery pack.
The Battery Management System (BMS) plays a pivotal role in monitoring cell conditions and implementing safety controls. It ensures optimal performance by balancing charge levels and preventing overcharging or deep discharging. Additionally, thermal management systems regulate operating temperatures, reducing the risk of overheating or thermal runaway. These components collectively influence the energy density, lifespan, and reliability of lithium-ion batteries.
1.2 How Lithium-ion Batteries Work
Lithium-ion batteries operate through a reversible electrochemical process. During charging, lithium ions move from the cathode to the anode through the electrolyte. This process stores energy within the battery. When discharging, the ions travel back to the cathode, releasing stored energy to power devices.
The movement of ions is facilitated by the separator, which ensures safe operation by preventing short circuits. The efficiency of this process depends on the quality of the materials used in the electrodes and electrolyte. By understanding this mechanism, you can appreciate how advancements in battery technology improve energy density and cycle life.
1.3 The Role of Chemistry in Battery Pack Performance
The chemistry of lithium-ion batteries directly impacts their performance and safety. For example, the choice of cell chemistry determines the energy density, cycle life, and thermal stability of the battery pack. Lithium-based materials like lithium cobalt oxide (LCO) and lithium iron phosphate (LiFePO4) offer distinct advantages for specific applications.
Ongoing research focuses on enhancing battery chemistry to meet the growing demands of industries such as transportation, robotics, and infrastructure. Improved material sourcing and end-of-life-cycle management contribute to sustainable production practices. By understanding the chemistry behind lithium-ion batteries, you can make informed decisions about their integration into your projects.
Part 2: Types of Lithium-ion Batteries for Electric Vehicles
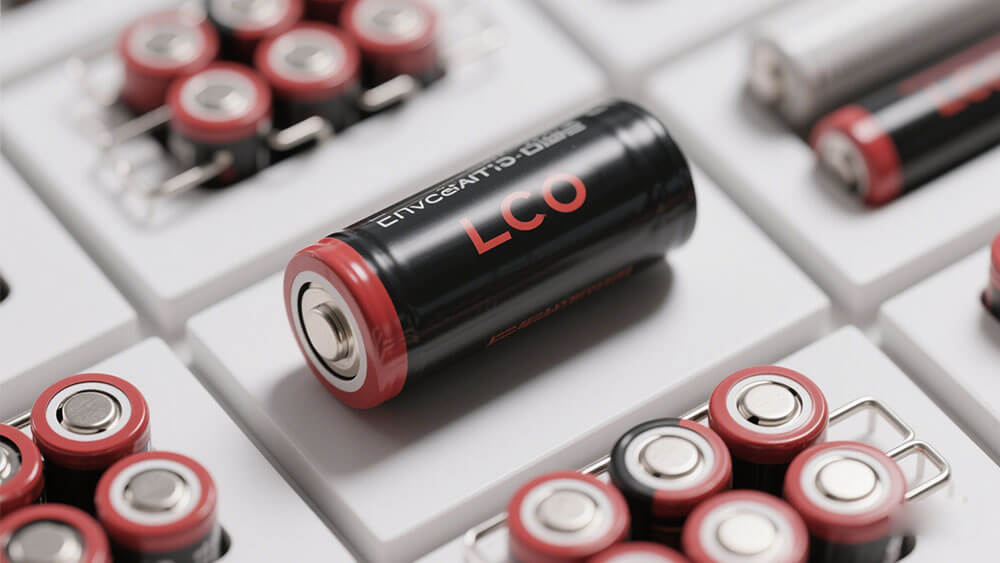
2.1 LCO Lithium Battery: High Energy Density, Limited Lifespan
Lithium cobalt oxide (LCO) batteries are known for their high specific energy, making them suitable for applications requiring compact designs and lightweight solutions. These batteries feature a platform voltage of 3.7V and an energy density ranging from 180 to 230 Wh/kg. Their ability to store significant energy in a small form factor makes them ideal for consumer electronics like smartphones and laptops.
However, the limited lifespan of LCO batteries, typically between 500 to 1,000 cycles, restricts their use in electric vehicles (EVs). The high cost and thermal instability further limit their adoption in large-scale applications. Despite these challenges, LCO batteries remain a niche choice for industries prioritizing high capacity over longevity.
2.2 LMO Lithium Battery: Safety and Thermal Stability
Lithium manganese oxide (LMO) batteries offer a balance between safety and performance. With a platform voltage of 3.7V and an energy density of 120 to 170 Wh/kg, these batteries deliver reliable energy output. Their low internal resistance enables fast charging and discharging, with current delivery reaching up to 20-30 Amps. This characteristic makes LMO batteries suitable for applications requiring quick energy replenishment.
The thermal stability of LMO batteries enhances their safety profile, reducing the risk of overheating. This feature makes them a preferred choice for security systems and infrastructure applications. In 2023, the market value of LMO batteries reached $7.24 billion, reflecting their versatility and growing demand across industries.
2.3 NMC Lithium Battery: Versatility and Balance
Lithium nickel manganese cobalt (NMC) batteries are among the most versatile options for EVs. These batteries combine high energy density (160 to 270 Wh/kg) with a platform voltage of 3.6-3.7V, offering an excellent balance between power and energy output. Automakers favor NMC batteries for their ability to optimize both range and performance.
NMC batteries excel in delivering longer driving ranges, with vehicles like the Tesla Model 3 achieving over 300 miles on a single charge. Their adaptability makes them suitable for premium cars, long-haul buses, and even electric trains. Ongoing research aims to enhance their lifespan and safety profile, ensuring they remain a top choice for EV manufacturers.
Feature | NMC Battery Advantages |
---|---|
Energy Density | Higher energy density for longer ranges |
Charging Speed | Faster charging speeds for convenience |
Long-Range Suitability | Ideal for long-distance travel with EVs |
2.4 LiFePO4 Lithium Battery: Cost-effectiveness and Longevity
Lithium iron phosphate (LiFePO4) batteries stand out for their cost-effectiveness and extended lifespan. With a platform voltage of 3.2V and an energy density of 100 to 180 Wh/kg, these batteries offer a cycle life of 2,000 to 5,000 cycles. This longevity significantly reduces replacement frequency, lowering the total cost of ownership.
LiFePO4 batteries are widely used in electric buses, trucks, and vans due to their safety and durability. Their compliance with safety standards minimizes risks, while their high energy efficiency reduces electricity costs. Compared to lead-acid batteries, LiFePO4 technology provides over 3,500 charge cycles while maintaining 80% capacity, making it a superior choice for long-term applications.
2.5 NCA Lithium Battery: High Energy Density for EVs
Nickel cobalt aluminum (NCA) batteries are designed for high-performance EVs. These batteries offer exceptional energy density, enabling vehicles to achieve extended ranges on a single charge. Their high specific energy makes them ideal for performance-oriented vehicles like sports cars and luxury EVs.
NCA batteries also support faster charging speeds, enhancing convenience for users. Studies have shown that these batteries exhibit minimal degradation under varying operating conditions, ensuring consistent performance over time. Their advanced chemistry makes them a reliable choice for demanding applications in the EV sector.
2.6 LTO Lithium Battery: Fast Charging and Durability
Lithium titanate oxide (LTO) batteries are renowned for their ultra-high cycle life and fast charging capabilities. With a platform voltage of 2.4V and an energy density of 60 to 90 Wh/kg, these batteries are built for durability. They can withstand 1,000 to 2,000 cycles, making them suitable for demanding operational requirements.
LTO batteries are commonly used in mining haul trucks and construction vehicles, where reliability is crucial. Their ability to charge quickly without compromising performance ensures uninterrupted operations. The robust design of LTO batteries makes them a dependable choice for industrial applications.
Battery Type | Key Characteristics | Applications |
---|---|---|
Lithium Iron Phosphate (LiFePO4) | Lower total cost of ownership, larger size allows more integration | Electric buses, trucks, vans |
Nickel-Manganese-Cobalt (NMC) | Highest energy densities, ideal for performance-oriented vehicles | Premium cars, long-haul buses/trucks, electric trains, eVTOL |
Lithium Titanate Oxide (LTO) | Ultra-high cycle life, suitable for demanding operational requirements | Mining haul trucks, construction vehicles |
Part 3: Comparison of Lithium-ion Battery Chemistries
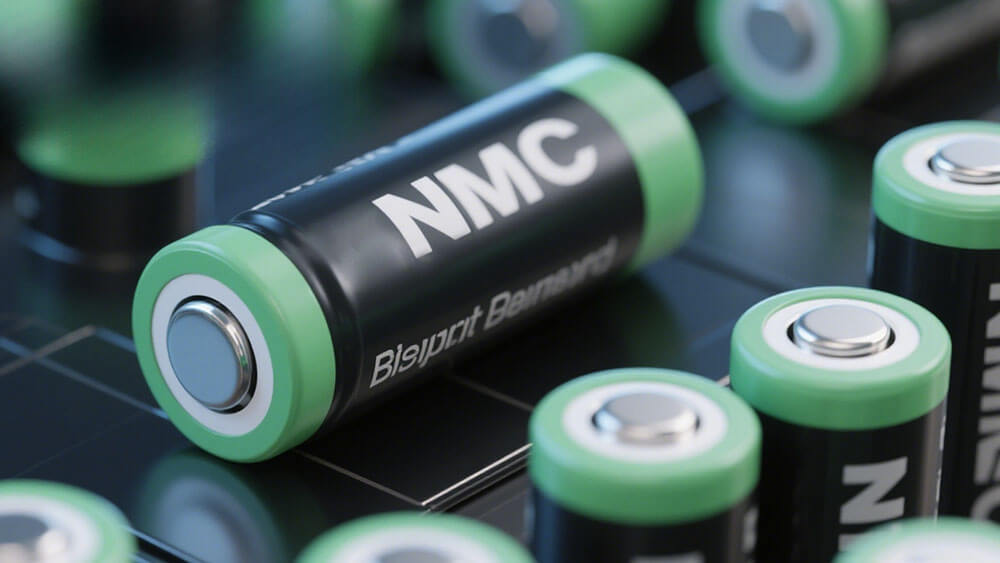
3.1 Energy Density and Range
Energy density plays a pivotal role in determining the range of electric vehicles (EVs). Higher energy density allows batteries to store more energy per unit weight, directly impacting the distance an EV can travel on a single charge. Among lithium-ion batteries, NCA and NMC chemistries stand out with energy densities of 200–260 Wh/kg and 160–270 Wh/kg, respectively. These batteries are ideal for long-range EVs, offering extended driving distances without increasing battery size or weight.
For instance, Tesla’s 4680 battery demonstrates how advancements in energy density can enhance range by up to 16%. Similarly, the CATL Qilin battery, with an energy density of 255 Wh/kg, enables lighter battery packs and greater range, making it a game-changer for EV manufacturers.
Battery Chemistry | Energy Density (Wh/kg) | Range Suitability |
---|---|---|
Lithium Nickel Cobalt Aluminum Oxide (NCA) | 200–260 | Long-range EVs |
Lithium Nickel Manganese Cobalt Oxide (NMC) | 160–270 | Versatile applications |
Lithium Iron Phosphate (LiFePO4) | 90–180 | Short to medium-range EVs |
Lithium Titanate (LTO) | 60–90 | Specialized industrial use |
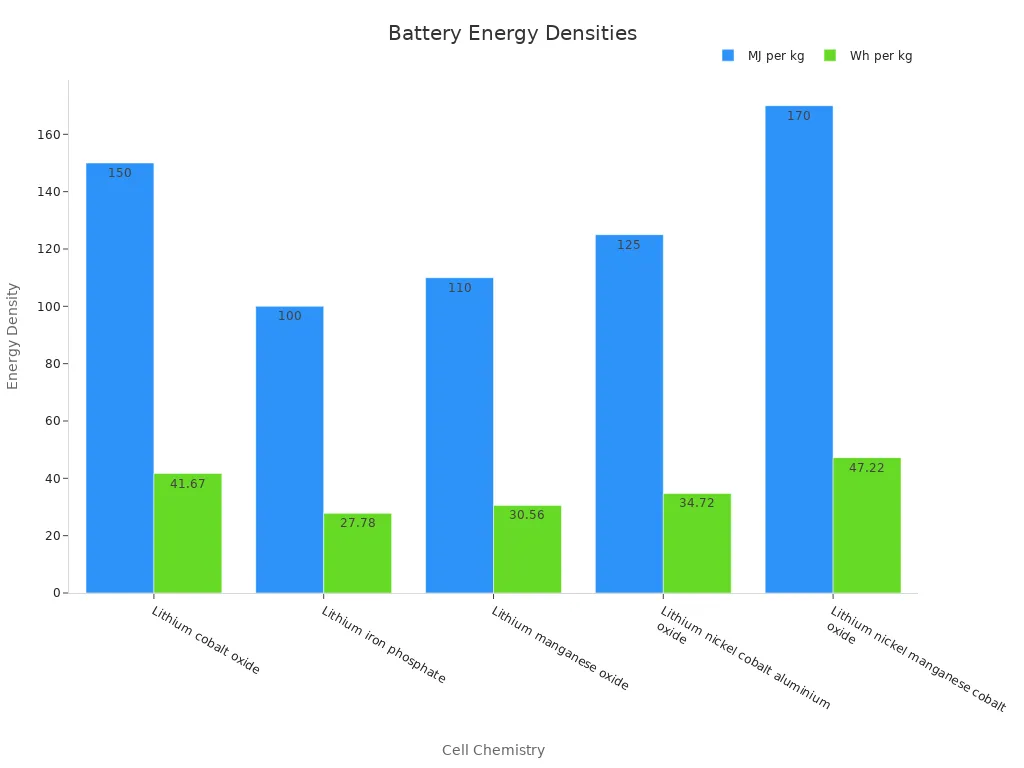
3.2 Cost and Scalability
Cost and scalability are critical factors in the adoption of lithium-ion batteries for EVs. LiFePO4 batteries are known for their cost-effectiveness, making them a popular choice for budget-friendly EVs and commercial vehicles. Their simpler manufacturing process and abundant raw materials contribute to lower production costs.
However, scaling up production presents challenges. The growing demand for lithium batteries has strained supply chains, leading to resource limitations and increased costs. For example, 76% of manufacturers report the need to upgrade or build new production lines to meet demand. Additionally, the push for sustainable practices adds pressure to adopt environmentally friendly production methods, further impacting scalability.
Challenge Type | Description |
---|---|
Growing Demand and Production Scalability | Limited resources and technical expertise hinder large-scale production. |
Environmental and Sustainability Pressures | Stricter regulations require manufacturers to adopt sustainable practices. |
Advances in Solid-State Battery Technology | Innovations are needed to transition from prototypes to mass production. |
3.3 Safety and Thermal Management
Safety remains a top priority in lithium-ion battery design. Thermal management systems play a crucial role in preventing overheating and thermal runaway incidents. LFP batteries excel in thermal stability, withstanding temperatures up to 270°C, making them one of the safest options for EVs. In contrast, NCA and LCO batteries, with thermal stability limits of 150°C, require advanced cooling systems to ensure safe operation.
Recent studies highlight innovations in thermal management. For example, Cao (2022) proposed a system combining phase change materials (PCMs) with liquid cooling, enhancing heat transfer efficiency. These advancements reduce the risk of thermal incidents and improve overall battery performance.
Battery Chemistry | Thermal Stability (°C) | Safety Features |
---|---|---|
Lithium Iron Phosphate (LFP) | 270 | High thermal stability |
Lithium Nickel Manganese Cobalt Oxide (NMC) | 210 | Moderate stability |
Lithium Nickel Cobalt Aluminum Oxide (NCA) | 150 | Requires advanced cooling |
Lithium Cobalt Oxide (LCO) | 150 | Limited thermal stability |
3.4 Lifespan and Degradation
The lifespan of lithium-ion batteries varies significantly across chemistries. LiFePO4 batteries lead the pack with a cycle life of 2,000–5,000 cycles, and some can even reach up to 6,000 cycles under optimal conditions. This longevity makes them ideal for applications requiring frequent charging, such as electric buses and delivery vehicles.
In contrast, NCA and LCO batteries have shorter lifespans, typically ranging from 500 to 1,000 cycles. These chemistries are better suited for applications prioritizing energy density over durability. Understanding degradation mechanisms, such as the loss of lithium inventory (LLI) and active material, is essential for optimizing battery performance and extending lifespan.
Battery Chemistry | Cycle Life | Applications |
---|---|---|
Lithium Iron Phosphate (LFP) | 2,000–5,000 | Electric buses, delivery vehicles |
Lithium Nickel Manganese Cobalt Oxide (NMC) | 1,000–2,000 | Passenger EVs |
Lithium Nickel Cobalt Aluminum Oxide (NCA) | 500 | High-performance EVs |
Lithium Cobalt Oxide (LCO) | 500–1,000 | Consumer electronics |
Understanding lithium-ion chemistry is essential for optimizing electric vehicle performance and sustainability. Recent advancements in battery technology have improved energy density, charging speeds, and efficiency, making electric vehicles more accessible. The price of lithium-ion battery packs dropped to USD 139/kWh in 2023, driving market growth. Innovations like organic materials for batteries promise sustainable solutions, aligning with industry trends. Businesses must invest in advanced lithium technologies to address cost, safety, and environmental challenges. Explore custom battery solutions tailored to your needs with Large Power.
FAQ
1. What factors influence the lifespan of a lithium-ion battery?
The lifespan depends on factors like charge cycles, operating temperature, and usage patterns. Proper maintenance and avoiding deep discharges can extend the battery’s life.
2. How do lithium-ion batteries ensure safety in electric vehicles?
Safety features include thermal management systems, separators to prevent short circuits, and Battery Management Systems (BMS) that monitor and regulate the battery’s performance.
3. Can lithium-ion batteries be customized for specific applications?
Yes, you can explore custom battery solutions tailored to your needs from Large Power. Industries like robotics, infrastructure, and medical devices benefit from specialized battery designs.