Contents
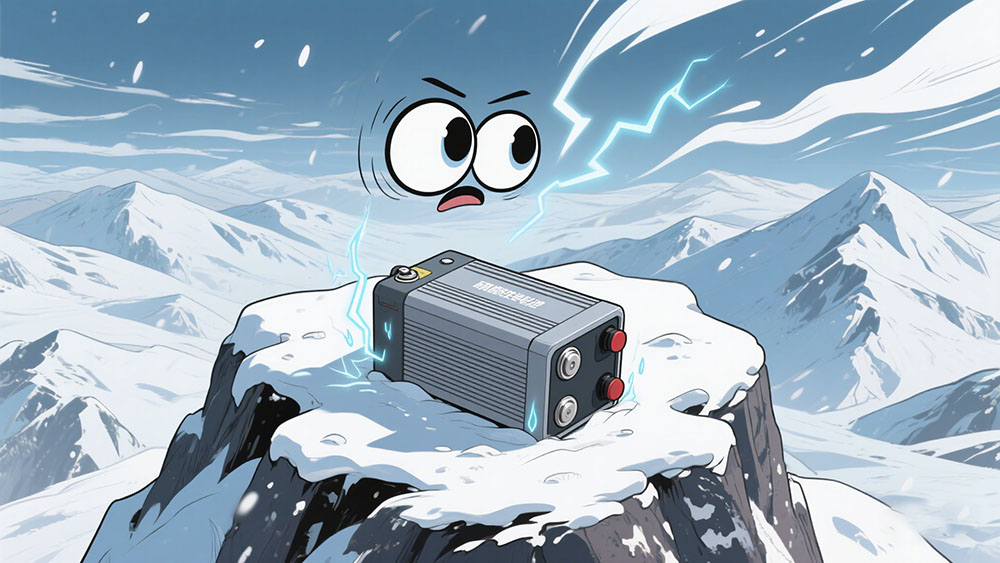
Low-temperature performance of LiFePO4 batteries plays a crucial role in industrial and energy storage applications. These batteries are widely used in sectors like infrastructure, medical, and robotics. You rely on their efficiency in extreme environments, yet cold conditions can lead to severe challenges:
The global demand for ultra low-temperature lithium batteries is rising, with the market projected to grow at a 9.8% CAGR, reaching USD 2.8 billion by 2032.
Lithium plating, which occurs when charging below 0°C, accelerates capacity fade and reduces efficiency.
Understanding these risks is vital to ensure reliable, long-lasting battery performance in harsh conditions.
Key Takeaways
Cold weather can make LiFePO4 batteries lose half their power. To keep them working well, use systems to manage heat.
Charging LiFePO4 batteries below freezing can cause damage. Always warm the battery first or use special charging tools.
Better electrolytes can help batteries work better in the cold. Look for ones that improve energy flow for steady power.
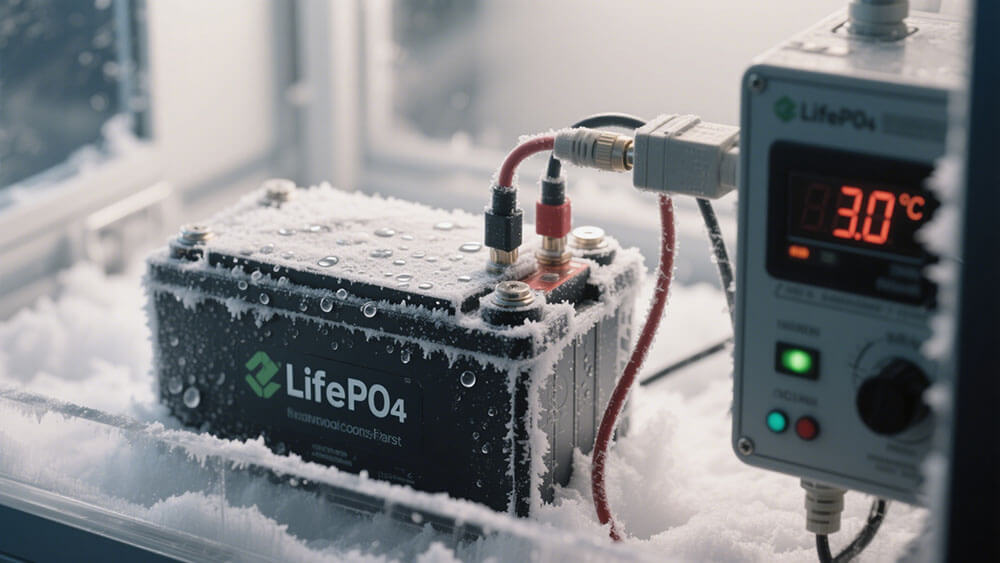
Part 1: Effects of Low-Temperature Performance of LiFePO4 batteries
1.1 Reduced Capacity and Efficiency in Cold Environments
Low temperatures significantly impact the capacity and efficiency of LiFePO4 batteries. When exposed to cold environments, these batteries experience a marked decline in their ability to store and deliver energy. For instance, at temperatures between -20°C and 0°C, capacity loss can reach up to 50%, while charging efficiency drops drastically, often leading to charging failures. Even in milder conditions, such as 0°C to 10°C, capacity loss ranges from 20% to 30%, and charging becomes slower, with potential risks of damage under high current.
Temperature Range | Effect on Capacity | Charging Efficiency |
---|---|---|
-20°C to 0°C | Significant capacity loss, up to 50% | Severely reduced, may lead to charging failure |
0°C to 10°C | Moderate capacity loss, around 20%-30% | Slower charging, potential damage at high current |
This reduction in performance stems from the slowed electrochemical reactions within the battery. As temperatures drop, the electrolyte becomes more viscous, reducing ionic conductivity and hindering the movement of lithium ions. This phenomenon is particularly concerning for applications in robotics, medical devices, and infrastructure, where consistent energy output is critical. To maintain optimal battery performance in such conditions, you must consider thermal management systems or alternative design strategies.
1.2 Increased Internal Resistance and Energy Loss
Cold temperatures also lead to increased internal resistance in LiFePO4 batteries. As the temperature drops, the resistance within the battery rises due to reduced ionic mobility and slower charge transfer reactions. This increase in resistance results in higher energy losses during both charging and discharging cycles.
For example, in industrial applications, such as transportation or security systems, higher internal resistance can cause voltage drops, reducing the overall efficiency of the battery pack. This inefficiency not only shortens the runtime of devices but also accelerates capacity degradation over time. The impact is particularly pronounced in consumer electronics, where users may notice shorter battery life and slower charging speeds in cold weather.
To mitigate these effects, you should explore optimized battery designs that minimize resistance, such as using advanced materials or incorporating preheating systems to maintain the battery’s internal temperature within an optimal range.
1.3 Risks of Lithium Plating When Charging LiFePO4 Below 32°F(0°C)
Charging at low temperatures poses a significant risk of lithium plating, a process where metallic lithium deposits on the anode surface instead of intercalating into the graphite structure. This phenomenon occurs because the reduced ionic conductivity and slower lithium-ion diffusion at low temperatures disrupt the balance between charge transfer and lithium-ion movement.
Charging the battery at low temperatures is likely to trigger lithium plating, which often leads to severe battery capacity fade. At −10°C, an 11.5 Ah lithium-ion cell showed a sharp increase in capacity degradation when the charge current exceeded 0.25C. Capacity loss reached 25% after just 40 cycles at a 0.5C charge rate. Even at 0°C, a single charge cycle at 1C caused a 3.6% irreversible capacity loss in a 7.5 Ah cell.
Lithium plating not only reduces the battery’s capacity but also increases the risk of internal short circuits, which can lead to thermal runaway and safety hazards. This issue is particularly critical in applications like medical devices and robotics, where reliability and safety are paramount.
To prevent lithium plating, you should avoid charging LiFePO4 batteries below 0°C or use specialized charging protocols designed for low-temperature conditions. Preheating systems can also help maintain the battery’s temperature above the critical threshold, ensuring safe and efficient charging.
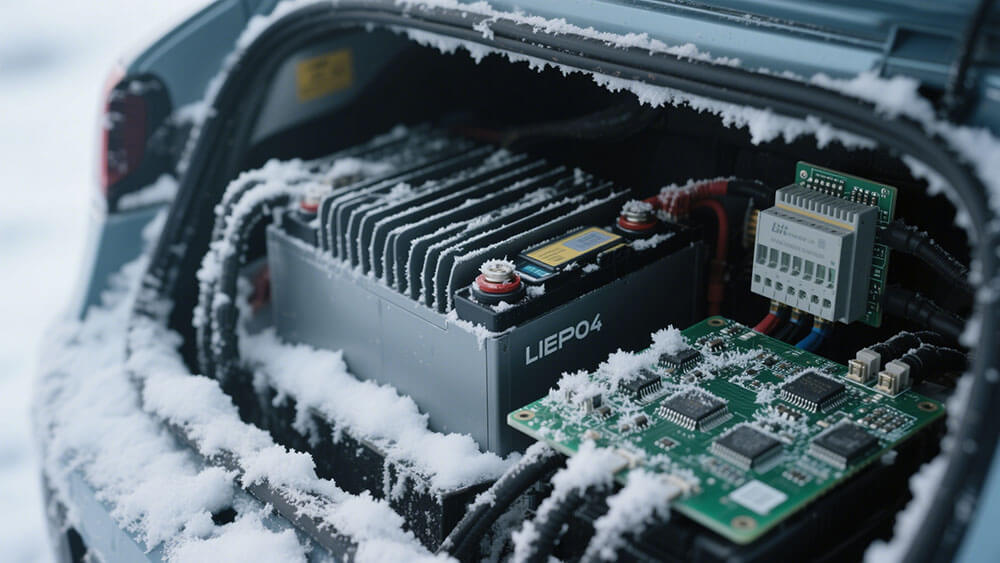
Part 2: Scientific Mechanisms Behind Low-Temperature Performance of LiFePO4 Batteries
2.1 Impact of Electrolyte Viscosity and Ionic Conductivity
The electrolyte in a LiFePO4 battery plays a critical role in facilitating lithium-ion migration between the electrodes. However, at low temperatures, the viscosity of the electrolyte increases significantly, which reduces its ionic conductivity. This change directly impacts the battery’s ability to deliver energy efficiently.
At 30°C, a electrolyte exhibits an ionic conductivity of 2.5 mS cm⁻¹. However, this value drops sharply to 0.22 mS cm⁻¹ at -20°C.
The Arrhenius equation effectively models this temperature-dependent behavior, showing that lower temperatures increase the activation energy required for ion transport.
A low activation energy of 0.33 eV indicates rapid lithium-ion migration under optimal conditions, but this advantage diminishes as the temperature decreases.
The reduced ionic conductivity at low temperatures hinders the movement of lithium ions, leading to slower charge transfer reactions and increased internal resistance. This phenomenon is particularly problematic in applications like medical devices, where consistent energy output is critical. To mitigate these effects, you can explore advanced electrolyte formulations designed to maintain higher ionic conductivity in cold environments.
2.2 Polarization and Its Effect on Discharge Voltage
Polarization is another key factor that affects the low-temperature performance of LiFePO4 batteries. It refers to the voltage difference between the theoretical and actual discharge voltage during operation. At low temperatures, polarization increases due to slower electrochemical reactions and higher charge transfer resistance.
Cathode (LiFePO4):
The olivine structure of LiFePO4 has narrow Li⁺ diffusion channels (1D pathways), leading to exponentially slower Li⁺ deintercalation at low temperatures.
Poor electronic conductivity (reliant on carbon coating) results in sharply increased charge transfer impedance (Rct).
Anode (Graphite):
Increased resistance to Li⁺ intercalation in graphite layers promotes lithium metal deposition (dendrites).
To address this issue, you should consider implementing preheating systems or optimizing the battery’s thermal management. These strategies can help maintain the internal temperature within an optimal range, reducing polarization and improving discharge performance.
2.3 Lithium-Ion Diffusion and Precipitation at Low Temperatures
Lithium-ion diffusion is a fundamental process in LiFePO4 batteries, enabling the transfer of lithium ions between the electrodes during charging and discharging. At low temperatures, this process becomes significantly slower due to the energy required for lithium ions to desolvate and embed in the graphite anode.
Studies show that at -2°C, charging rates exceeding C/2 lead to increased lithium precipitation, with up to 9% of capacity lost at a 1C rate.
Neutron diffraction studies reveal that phase changes in the negative electrode during low-temperature charging differ based on charging rates, affecting lithium intercalation.
This hindered diffusion results in increased electrochemical polarization and the precipitation of metallic lithium on the anode surface. Such precipitation not only reduces the battery’s capacity but also poses safety risks, including internal short circuits and thermal runaway.
To prevent these issues, you should avoid charging LiFePO4 batteries below 0°C or use specialized charging protocols designed for low-temperature conditions. Advanced materials and electrolyte formulations can also enhance the lithium-ion migration rate, ensuring better performance in cold environments.
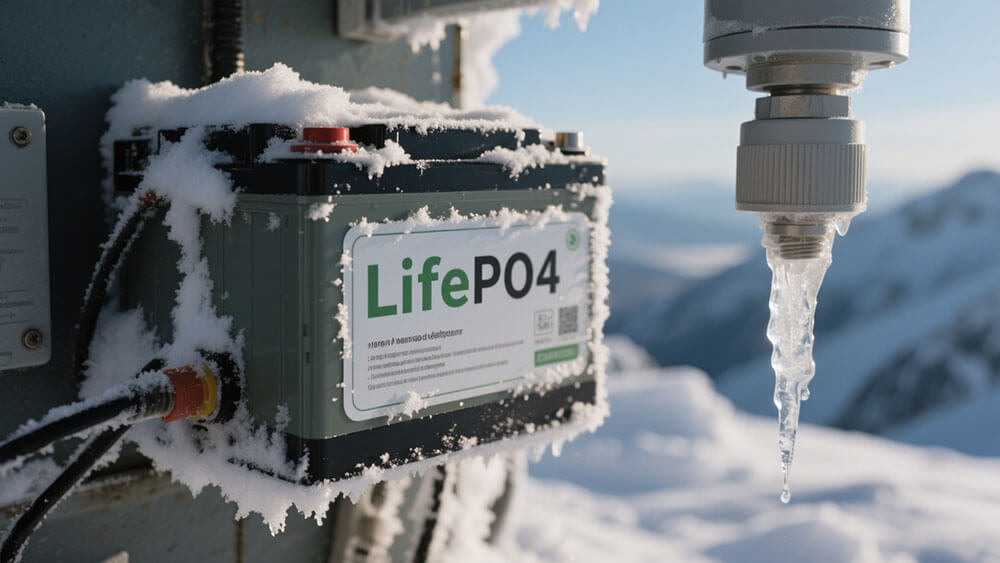
Part 3: Strategies to Mitigate Low-Temperature Performance Issues
3.1 Preheating Systems and Thermal Management for Battery Packs
Preheating systems and thermal management solutions are essential for maintaining the performance of LiFePO₄ batteries in cold environments. These systems ensure the battery operates within its optimal temperature range, typically 25 to 35°C (77 to 95°F). Battery Thermal Management Systems (BTMS) use active, passive, or hybrid heat transfer methods to regulate temperature effectively.
Method | Description |
---|---|
Active Heat Transfer | Uses external energy to regulate temperature |
Passive Heat Transfer | Relies on natural heat flow |
Hybrid | Combines active and passive methods |
For cold weather applications, BTMS can heat the coolant to prevent the battery from freezing. This approach minimizes the risk of lithium plating and capacity loss. However, designing these systems requires balancing thermal conductivity, heat capacity, and power consumption. For example, systems with higher thermal conductivity outperform air-based solutions but involve greater design complexity.
3.2 Optimized Battery Pack Design for Cold Weather Applications
Optimized battery pack designs play a critical role in enhancing the performance of lithium-ion batteries in cold conditions. Incorporating cold plates into the design improves thermal management by transferring heat efficiently. This prevents overheating and ensures consistent performance.
Cold plates enhance heat transfer in low-temperature environments.
Optimizing flow dynamics and cooling channel geometry improves thermal efficiency.
Material selection impacts the longevity and reliability of the battery pack.
These design principles, backed by numerical simulations, demonstrate significant improvements in battery performance. For industrial applications like transportation and infrastructure, such designs ensure reliability and extend battery life.
3.3 Advanced Electrolyte Formulations to Enhance Low-Temperature Performance
Advanced electrolyte formulations address the challenges of reduced ionic conductivity and lithium-ion mobility in cold environments. Research highlights the effectiveness of binary solvent systems and multifunctional additives in improving performance.
A study revealed that a binary solvent system with strong and weak solvating solvents significantly enhanced ionic conductivity. The addition of perfluoroalkylsulfonyl quaternary ammonium nitrate (PQA-NO3) improved lithium-ion battery performance at ultra-low temperatures. The Li||NMC811 full cell maintained 48.1% of its room temperature capacity at −85°C.
Electrolytes with optimized components, such as ethylene carbonate and lithium hexafluorophosphate (LiPF6), exhibit higher conductivity at low temperatures. These formulations reduce internal resistance and improve charge transfer, making them ideal for applications in robotics and medical devices.
Low temperatures significantly affect LiFePO4 batteries, causing reduced capacity, increased internal resistance, and lithium plating risks. Understanding the scientific mechanisms behind these challenges allows you to implement effective strategies, such as advanced electrolyte formulations and optimized thermal management systems.
Adopting these solutions ensures reliable performance in critical applications like medical devices, robotics, and infrastructure. Continued innovation in low-temperature battery technologies will drive sustainability and efficiency in the energy sector. Explore custom solutions with Large Power to optimize your battery systems for cold environments.
FAQ
1. What happens if you charge a LiFePO4 battery below 0°C?
Charging below 0°C can cause lithium plating, leading to capacity loss and safety risks. Always preheat the battery or use specialized charging protocols.
2. How can you improve the performance of LiFePO4 batteries in cold weather?
You can use preheating systems, advanced electrolyte formulations, or optimized battery pack designs to enhance performance and prevent capacity loss in low temperatures.
3. Why do LiFePO4 batteries lose capacity in cold environments?
Low temperatures increase internal resistance and reduce ionic conductivity, slowing electrochemical reactions. This limits the battery’s ability to store and deliver energy efficiently.