Contents
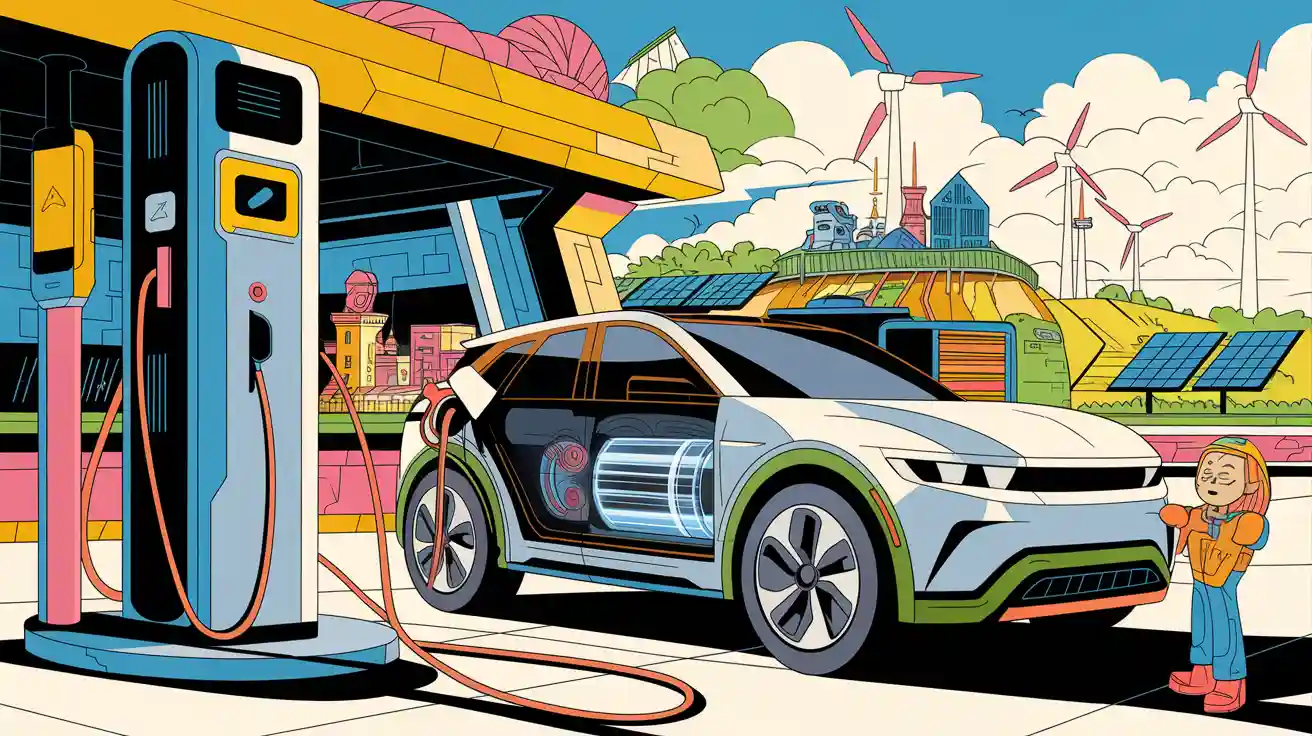
Lithium-ion batteries have revolutionized electric vehicles, driving advancements in range, efficiency, and adoption. Their energy density, ranging from 100 Wh/kg to 270 Wh/kg, enables EVs to achieve 150–400 miles per charge. By 2030, EV sales will comprise 30% of the global market, underscoring the transformative impact of electric vehicles lithium battery technology.
In 2020, the global EV industry generated $176.1 billion in revenue, projected to reach $561.4 billion by 2023, reflecting sustained demand for EVs.
Key Takeaways
Lithium-ion batteries have gotten much better. Their energy storage grew from 100-120 Wh/kg in 1991 to over 270 Wh/kg now. This helps electric cars go farther.
Solid-state batteries are safer and store more energy. They can reach up to 500 Wh/kg and last 8,000-10,000 charges. These could be the future of batteries.
New ideas like sodium-ion batteries and fast charging are solving material problems. They also make charging quicker, making electric cars easier to use.
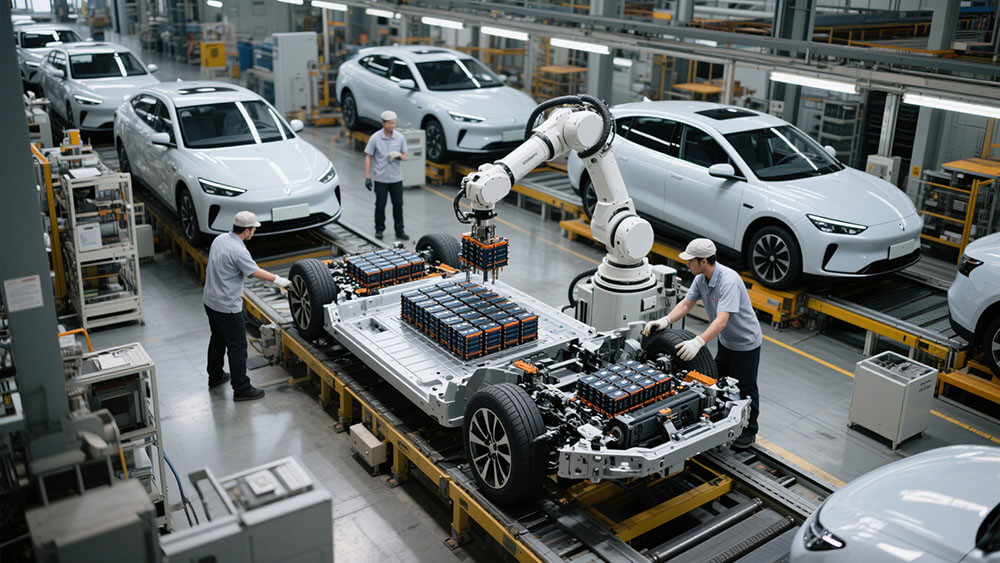
Part 1: The History of Electric Vehicles Lithium Battery
1.1 Early developments in lithium-ion batteries
The journey of lithium-ion batteries began in the early 1990s when they were first commercialized. These batteries quickly gained attention for their high energy density and lightweight design, making them ideal for portable electronics. Early experimental efforts, such as those at the University of Michigan’s Battery Lab, played a pivotal role in advancing this technology. Established in collaboration with Ford in 2012, the lab has since facilitated numerous innovations, including large-scale cell builds and alternative chemistries. These foundational developments laid the groundwork for integrating lithium-ion batteries into electric vehicles.
1.2 Key milestones in EV battery technology
The evolution of electric vehicles lithium battery technology is marked by significant milestones. Since the introduction of the first commercial lithium-ion battery in 1991, energy density has increased from 100-120 Wh/kg to over 300 Wh/kg today. The cost of battery packs has also dropped dramatically, from $668/kWh in 2013 to $137/kWh in recent years, a reduction of nearly 80%.
These milestones underscore the rapid progress in battery technology, enabling electric vehicles to achieve longer ranges and improved performance.
1.3 The role of lithium-ion batteries in shaping the EV market
Lithium-ion batteries have been instrumental in the growth of the electric vehicle market. By 2018, global lithium-ion battery sales reached 160 GWh, with 44% allocated to electric vehicles and e-buses. This growth reflects the increasing demand for cleaner transportation solutions. The affordability and efficiency of lithium-ion batteries have made them a cornerstone of the EV industry, driving adoption rates and market expansion. For instance, electric car sales reached 2.1 million in 2019, accounting for 2.6% of global car sales. As governments and industries prioritize sustainable transportation, lithium-ion batteries continue to play a critical role in achieving longer driving ranges and enhanced EV performance.
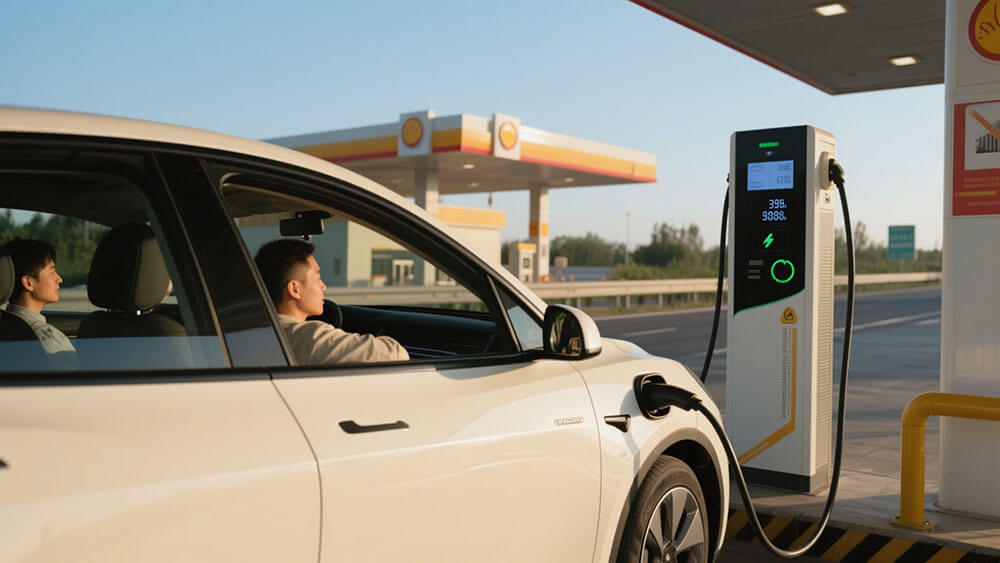
Part 2: Latest Advancements in Lithium-Ion Battery Technology
2.1 Solid-state batteries: A breakthrough in energy density and safety
Solid-state batteries represent a transformative leap in lithium-ion technology. By replacing flammable liquid electrolytes with solid, non-flammable materials, these batteries significantly enhance safety. This innovation reduces the risk of thermal runaway and fire, addressing one of the most critical concerns in electric vehicle (EV) applications. Furthermore, solid-state batteries offer an impressive energy density of 300–500 Wh/kg, enabling EVs to achieve longer ranges and extended lifecycles. These batteries can endure 8,000 to 10,000 charge cycles, far surpassing the 500 to 5,000 cycles of traditional lithium-ion batteries.
The integration of solid-state batteries into EVs not only improves performance but also aligns with sustainability goals by reducing material dependency and enhancing recyclability. As the industry continues to innovate, solid-state technology is poised to redefine the future of EV battery systems.
2.2 Emerging alternatives: Quasi-solid-state and sodium-ion batteries
Quasi-solid-state and sodium-ion batteries are gaining traction as promising alternatives to traditional lithium-ion batteries. Quasi-solid-state batteries combine the benefits of solid and liquid electrolytes, offering improved energy density and safety. These batteries bridge the gap between current lithium-ion technology and fully solid-state systems, making them a viable option for near-term EV applications.
Sodium-ion batteries, on the other hand, leverage abundant and cost-effective sodium resources, addressing the material dependency challenges associated with lithium and cobalt. Although their energy density (100–150 Wh/kg) currently lags behind lithium-ion batteries, ongoing research aims to close this gap. These alternatives highlight the industry’s commitment to diversifying battery chemistries and reducing reliance on critical materials. For a deeper understanding of sustainable practices in battery production, visit at Large Power.
2.3 Fast-charging technologies: Reducing downtime for EVs
Fast-charging technologies are revolutionizing the EV landscape by minimizing charging times and enhancing user convenience. Advanced lithium-ion battery systems now support rapid charging, enabling EVs to achieve an 80% charge in as little as 15 minutes. The table below illustrates the performance metrics of various fast-charging technologies:
EV Model | Charging Time (to 80%) | Distance Travelled | Charging Technology |
---|---|---|---|
Tesla | 15 minutes | 200 miles | Supercharger network |
Porsche Taycan | 5.5 minutes | 100 kilometers | 800-volt charging stations |
QuantumScape | 15 minutes | 10-80% charge | Advanced lithium-ion battery |
These advancements not only reduce downtime but also enhance the practicality of EVs for long-distance travel. By integrating fast-charging capabilities, manufacturers address one of the primary barriers to EV adoption, ensuring a seamless user experience.
2.4 Graphene and silicon anodes: Enhancing energy storage capabilities
The use of graphene and silicon anodes in lithium-ion batteries marks a significant advancement in energy storage technology. Graphene’s exceptional conductivity and mechanical strength enhance battery performance, while silicon anodes offer a theoretical capacity of ~4,200 mAh/g, far exceeding the ~372 mAh/g of traditional graphite anodes. The table below highlights recent research findings:
These innovations improve energy density and cycle life, making lithium-ion batteries more efficient and durable. As a result, EVs can achieve longer ranges and better performance. The integration of graphene and silicon anodes underscores the industry’s focus on pushing the boundaries of lithium-ion technology.
For customized battery solutions tailored to your specific needs, explore our custom battery solutions.
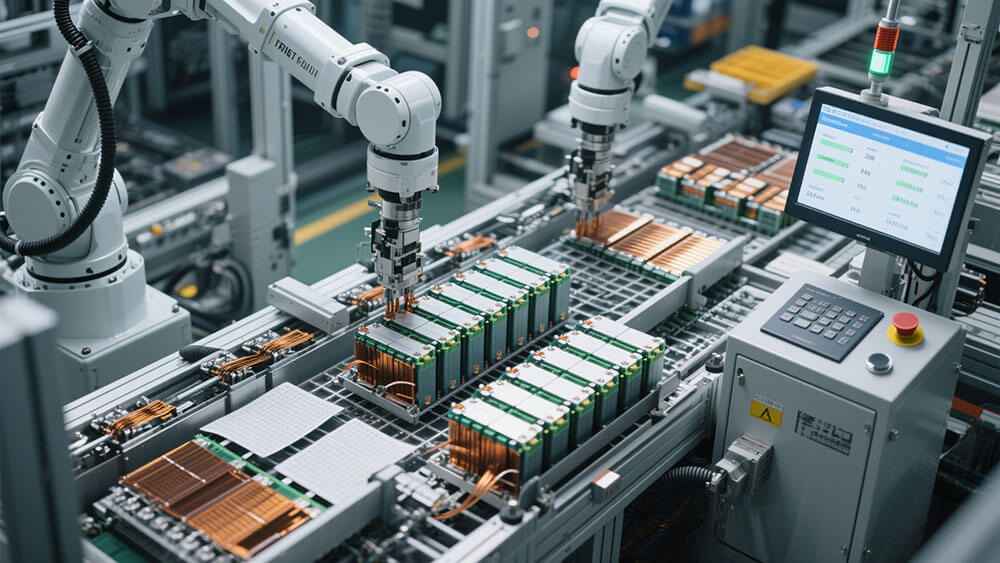
Part 3: Challenges in Lithium-Ion Battery Production
3.1 Material dependency: Cobalt, lithium, and sustainable alternatives
The production of lithium-ion batteries heavily relies on critical materials like cobalt and lithium. These materials are essential for achieving the high energy density and performance required in electric vehicles. However, their limited availability and uneven geographical distribution pose significant challenges. For instance, over 68% of global cobalt refining capacity and 72% of lithium refining capacity were controlled by Chinese companies in 2022. This concentration creates supply chain vulnerabilities and increases the risk of price volatility.
Price fluctuations further complicate the situation. In 2022, cobalt and nickel price surges led to a 7% increase in battery prices, directly impacting the cost of electric vehicles. To mitigate these risks, the industry is exploring sustainable alternatives such as sodium-ion batteries and recycling initiatives. These efforts aim to reduce dependency on scarce materials while ensuring the scalability of battery technology.
3.2 Environmental and ethical concerns in sourcing
The extraction and processing of materials like lithium, cobalt, and nickel significantly contribute to the environmental footprint of lithium-ion batteries. Mining operations often result in habitat destruction, water pollution, and high carbon emissions. Approximately 40% of the climate impact from lithium-ion battery production arises from the mining and processing of these minerals.
Ethical concerns also play a critical role. Cobalt mining, particularly in the Democratic Republic of Congo, has been linked to child labor and unsafe working conditions. These issues highlight the need for transparent supply chains and responsible sourcing practices. Recycling offers a potential solution, but only 5% of lithium-ion batteries are recycled globally, compared to 99% of lead-acid batteries in the U.S. The lack of standardization in battery design and the hazardous nature of dismantling processes further complicate recycling efforts.
Addressing these challenges requires a multi-faceted approach, including stricter regulations, technological innovation, and industry collaboration. For more information on conflict-free sourcing, explore this statement.
3.3 Cost and scalability in battery manufacturing
The high energy intensity of lithium-ion battery production presents a significant barrier to cost reduction. Manufacturing these batteries is three times more energy-intensive than producing internal combustion vehicle batteries. This energy demand not only increases production costs but also contributes to the overall carbon footprint of electric transport.
Scalability remains another critical challenge. As demand for electric vehicles grows, manufacturers must scale production without compromising quality or sustainability. However, the current recycling infrastructure is insufficient to meet this demand. It is often more cost-effective for manufacturers to source newly mined materials than to recycle existing ones, further exacerbating material dependency.
To overcome these obstacles, companies are investing in advanced manufacturing techniques and exploring alternative chemistries like LiFePO4 Lithium batteries. These innovations aim to enhance efficiency and reduce costs, ensuring the long-term viability of battery technology. For tailored solutions to meet your specific needs, explore Large Power’s custom battery solutions.
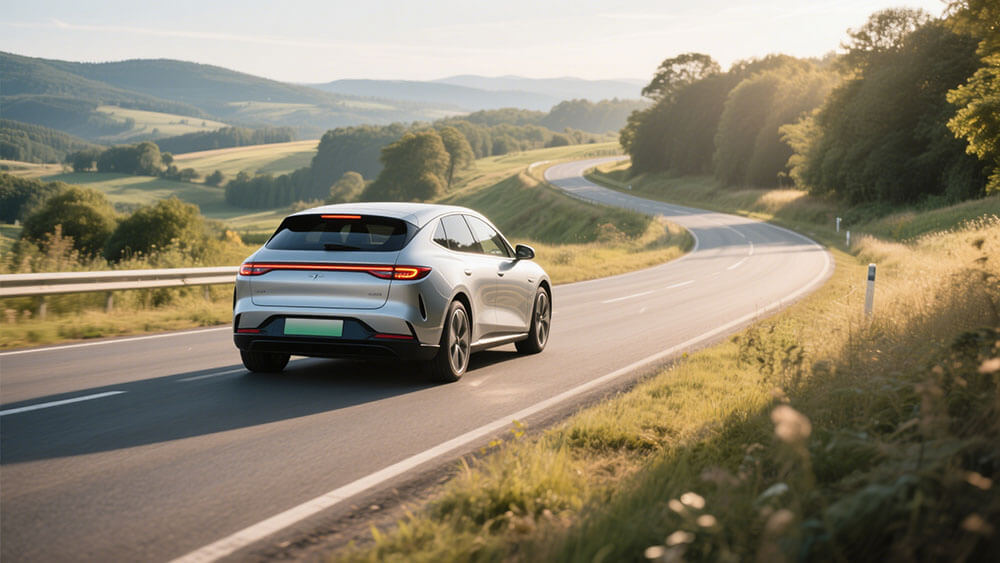
Part 4: Future Trends in Battery Technology for Electric Vehicles
4.1 Regulatory changes and their influence on battery innovation
Regulatory frameworks are reshaping the landscape of battery technologies for electric vehicles. As governments worldwide push for stricter emissions standards and sustainability goals, you will see increased emphasis on recycling materials from end-of-life batteries. This approach reduces reliance on traditional mining, which often faces geopolitical risks, and supports a circular economy.
Key Areas of Focus | Insights |
---|---|
Electric Vehicle Sales | Analysis of sales trends and market share in the EV sector. |
Technological Advancements | Examination of innovations in battery technology and charging systems. |
Growth Projections | Forecasts on the future growth of the electric vehicle market. |
These regulatory changes drive advancements in battery technologies, encouraging manufacturers to innovate in areas like energy density and charging efficiency. For example, solid-state batteries are emerging as a significant innovation, offering enhanced safety and performance.
4.2 AI and data-driven optimization of battery performance
Artificial intelligence (AI) is revolutionizing how you optimize battery performance. By analyzing large volumes of usage data, AI identifies patterns that inform better battery management strategies. Predictive maintenance powered by AI helps you detect potential issues before they occur, prolonging battery lifespan and reducing downtime.
AI predicts battery health and optimizes charging methods, enhancing overall performance.
Engineering tests benefit from predictive analytics, streamlining testing processes and improving resource allocation.
Insights extracted from test data lead to better performance outcomes and cost efficiency.
AI-driven solutions also enable you to optimize energy storage systems, ensuring high-capacity battery designs meet the demands of modern electric vehicles. For tailored solutions to integrate AI into your battery systems, explore our custom battery solutions.
4.3 The potential of lithium-sulfur and lithium-metal batteries
Lithium-sulfur and lithium-metal batteries represent the next frontier in energy storage for electric vehicles. These alternatives offer higher theoretical capacities and energy densities, with lithium-sulfur batteries exceeding 500 Wh/kg and potentially approaching 1,000 Wh/kg. Their lower material costs and abundant sulfur sources make them attractive for weight-sensitive applications.
Stabilization of the sulfur cathode improves cycle life and resolves capacity fade issues.
Lithium-metal batteries deliver enhanced safety features and scalability for practical applications.
These technologies are ideal for high-capacity battery designs, addressing the growing demand for efficient energy storage solutions.
The advancements in battery technologies, including lithium-sulfur and lithium-metal systems, position you to meet the evolving needs of the electric vehicle market.
The evolution of lithium-ion batteries has transformed electric vehicles.
Over 30 years, costs have dropped by 97%, while energy density has significantly improved.
By 2030, the global battery market will reach $399.45 billion, driven by sustainability initiatives.
Future innovations will ensure scalable growth, supporting a cleaner, more efficient transportation ecosystem.
For tailored solutions, explore custom battery solutions.
FAQ
1. What makes lithium-ion batteries ideal for electric vehicles?
Lithium-ion batteries offer high energy density, long cycle life, and lightweight design. These features make them efficient and reliable for EV applications.
2. How do solid-state batteries differ from traditional lithium-ion batteries?
Solid-state batteries replace liquid electrolytes with solid materials. This change improves safety, energy density, and cycle life, making them a promising innovation for EVs.
3. Can Large Power provide customized battery solutions for specific industries?
Yes, Large Power specializes in tailored battery solutions for industries like medical, robotics, and infrastructure.