Contents
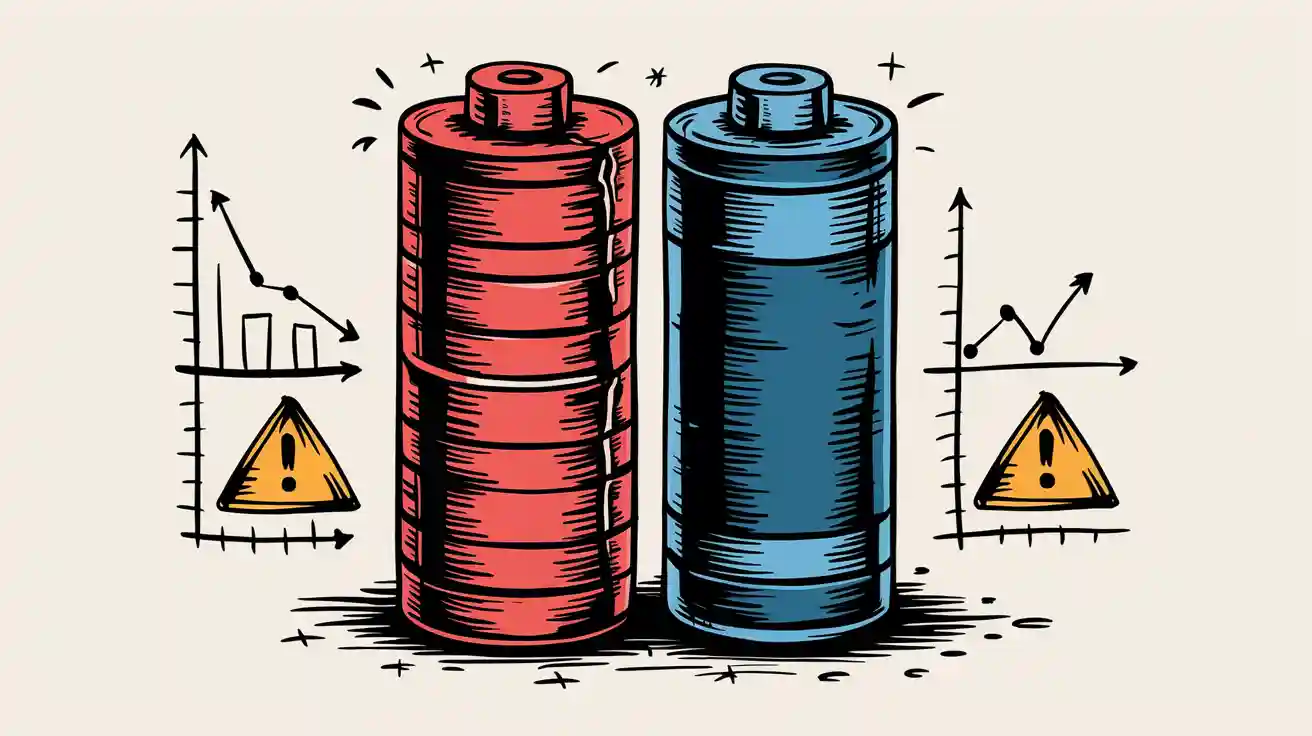
The gradual degradation of lithium battery impacts both performance and safety significantly. As batteries age, side reactions and material degradation reduce their energy storage capacity and increase internal resistance. Over time, this leads to slower charging, higher heat generation, and safety risks like overheating. Experimental data from over 3 billion measurements highlights how capacity retention curves decline due to first-life degradation. For industrial applications, understanding and addressing first life SOH is critical to ensure protection and reliable performance. To mitigate high temperature effects, you must avoid extreme temperatures and implement strategies to extend battery life.
Key Takeaways
Check battery health often. Watch for signs like losing charge or harder charging to spot aging early.
Charge the right way. Keep the battery between 20% and 80% to lower stress and make it last longer.
Keep the temperature steady. Use lithium batteries in 15-35°C to avoid damage and stay safe.
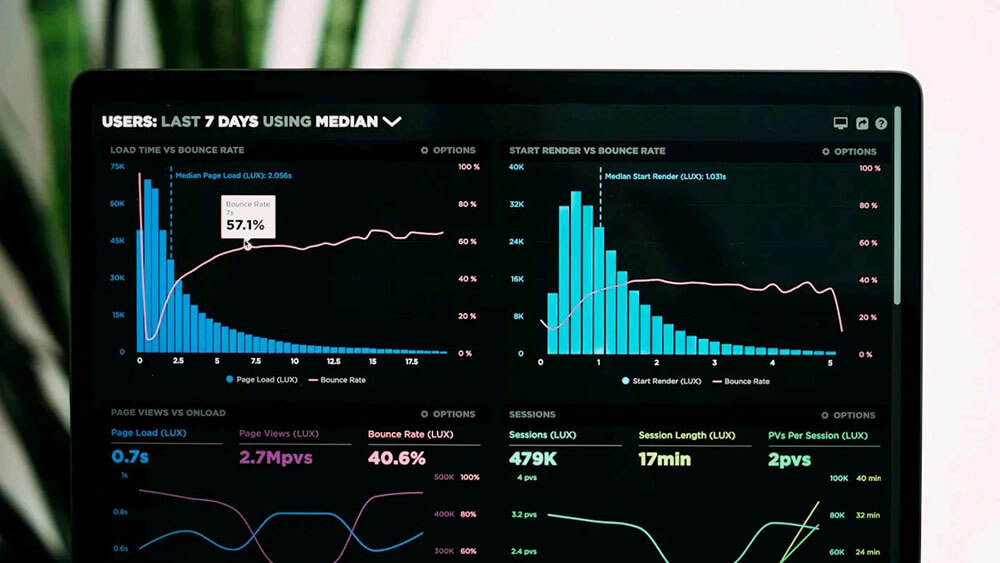
Part 1: Understanding Lithium-Ion Battery Aging
1.1 What Is Battery Aging?
Battery aging refers to the gradual decline in a lithium-ion battery’s performance and capacity over time. This process occurs due to various degradation mechanisms that impact the battery’s ability to store and deliver energy efficiently. Two primary types of aging are calendar aging and cycle aging. Calendar aging happens when a battery loses capacity over time, even without active use, often influenced by high temperatures and a high state of charge. On the other hand, cycle aging results from repeated charging and discharging cycles, which accelerate wear on the battery’s internal components.
Type of Aging | Description |
---|---|
Calendar Aging | Capacity loss of the battery over time without cycling, influenced by temperature and state of charge. |
Cycle Aging | Capacity loss due to repeated charge and discharge cycles at different temperatures. |
Understanding these processes is crucial for effective degradation analysis and improving the state of health of lithium-ion batteries.
1.2 Why Lithium-Ion Battery Aging Matters
Lithium-ion battery degradation directly affects performance, safety, and lifespan. As batteries age, their state of health declines, leading to reduced energy efficiency and increased internal resistance. This can result in overheating, which raises the risk of thermal runaway or even explosion. For industrial applications, where reliability is critical, understanding degradation mechanisms is essential. A comprehensive degradation analysis helps identify degradation causes and optimize battery usage to extend lifespan and ensure safety.
1.3 Key Indicators of Battery Degradation
Monitoring key indicators of battery degradation is vital for maintaining performance and safety. These indicators include:
Capacity loss: A noticeable reduction in the battery’s ability to store energy.
Increased internal resistance: This leads to power decline and heat generation during operation.
Voltage irregularities: Fluctuations in voltage during charging or discharging cycles.
Researchers have developed advanced datasets and statistical models to study these indicators. For instance, a dataset with 279 cells and 71 distinct aging conditions has been used to uncover hidden dependencies in degradation mechanisms. These insights are critical for improving battery performance and predicting the state of health over time.
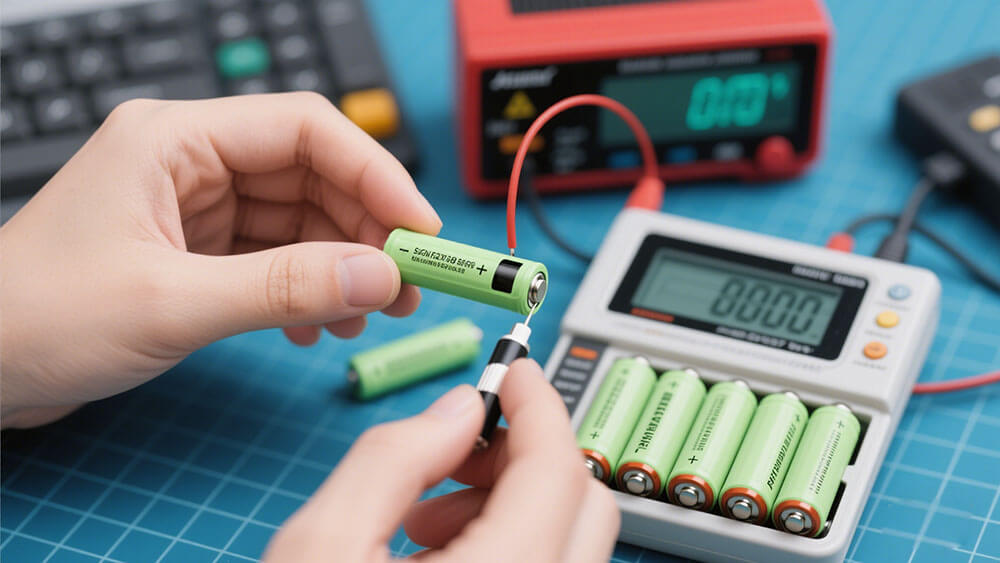
Part 2: Causes of Lithium-Ion Battery Degradation
2.1 Chemical Reactions and Electrolyte Breakdown
Chemical reactions within lithium-ion batteries are a primary root cause of degradation. Over time, these reactions lead to the breakdown of the electrolyte and the formation of a solid electrolyte interphase (SEI) layer. While the SEI layer protects the anode, its growth consumes lithium ions, reducing the battery’s capacity. The extremely lean electrolytic testing (ELET) methodology has quantified how electrolyte decomposition impacts battery lifespan. This analysis highlights that SEI growth accelerates during cycles where a significant drop in capacity occurs, directly affecting performance.
Parameter | Description |
---|---|
SEI film thickness | Approximately linearly related to the number of cycles, indicating its role in capacity decay. |
Lithium plating thickness | Rapidly stabilizes in early cycles, correlating with performance decline in those cycles. |
Discharge capacity decay | Shows significant differences between early and late cycles, indicating lithium-ion loss dynamics. |
Charge rate effects | High charge rates lead to increased lithium plating, while medium rates show no plating. |
Temperature effects | Low temperatures exacerbate lithium plating, impacting degradation rates. |
You can mitigate these effects by optimizing charging protocols and using advanced electrolyte formulations. These strategies help slow down SEI growth and extend the lifespan of lithium-ion batteries.
2.2 Impact of Temperature on Battery Aging
The impact of temperature on lithium-ion battery aging cannot be overstated. High temperatures accelerate chemical reactions, leading to faster electrolyte breakdown and SEI growth. Conversely, low temperatures increase lithium plating, which reduces capacity and raises safety risks. Controlled experiments have demonstrated that degradation rates rise significantly with temperature. For instance, at 30°C, the degradation rate increases sharply, with half of the battery’s capacity lost within a few cycles.
At 35°C, battery cycle life estimates range from over 3,000 cycles to closer to 2,000 cycles.
At 25°C, the optimistic estimate is around 3,000 cycles.
A pessimistic estimate at 30°C is 2,000 cycles, while an optimistic estimate is 4,000 cycles.
The lifetime of LFP cells declines more rapidly between 15°C and 45°C compared to 45°C and 60°C.
Maintaining optimal operating temperatures is crucial. You should implement thermal management systems to prevent overheating and ensure consistent performance.
2.3 Effects of Charging and Discharging Cycles
Charging and discharging cycles are another root cause of degradation in lithium-ion batteries. Each cycle contributes to wear and tear on the battery’s internal components. Depth of discharge (DOD), charging rates, and frequency of cycles all play a role in determining the degradation rate. For example, high charge rates can lead to lithium plating, while overcharging or undercharging causes stress on the electrodes.
Internal factors like material composition influence performance during cycles.
External factors such as temperature and humidity can cause thermal stress or corrosion.
Improper current and voltage levels during charging may result in overcharging or undercharging.
Frequent deep discharges accelerate wear, reducing the battery’s lifespan.
To minimize these effects, you should adopt best practices for charging, such as avoiding extreme DOD and using smart chargers that regulate current and voltage.
2.4 Role of Material Quality and Manufacturing Processes
Material quality and manufacturing processes significantly influence the degradation rate of lithium-ion batteries. Defects in materials or inconsistencies during production can lead to uneven performance and faster aging. Advanced techniques like in-line mass profilometry and Raman spectroscopy have improved quality control by identifying defects and ensuring uniformity.
Technique | Description | Impact on Battery Degradation Rates |
---|---|---|
In-line Mass Profilometry | Measures mass loading of active material on electrodes in real-time. | Reduces scrap rates and ensures uniformity, impacting degradation. |
Raman Spectroscopy | Analyzes defect concentrations in coatings. | Helps identify defects that can enhance electrochemical properties. |
Improved inspection methods, such as 3D X-ray imaging, also play a critical role in detecting flaws that could compromise battery performance. By investing in high-quality materials and advanced manufacturing techniques, you can significantly reduce the root cause of degradation and enhance the reliability of lithium-ion batteries.
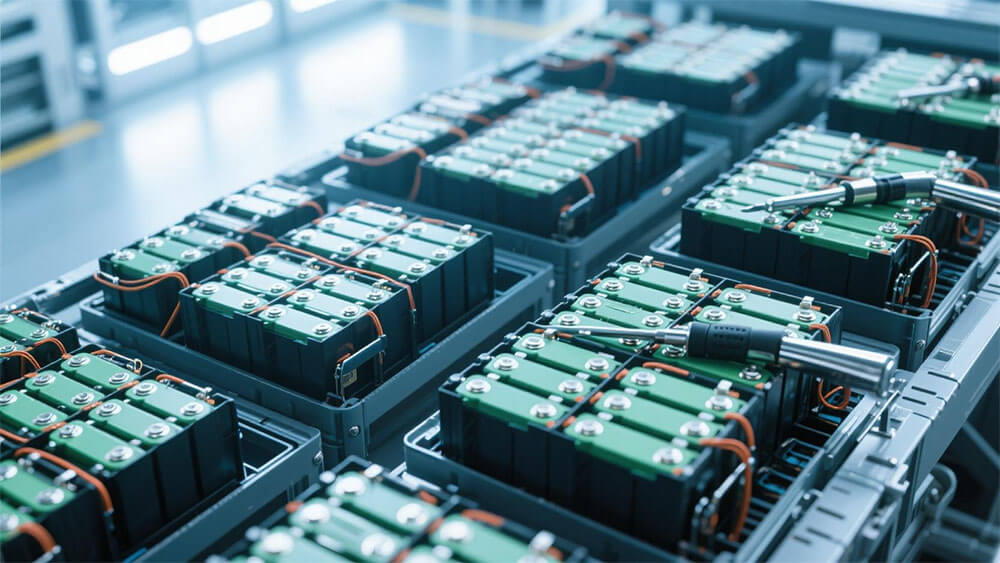
Part 3: Effects of Battery Aging on Performance and Safety
3.1 Capacity Loss and Reduced Energy Efficiency
As lithium-ion batteries age, capacity loss becomes one of the most noticeable effects. This reduction in capacity directly impacts energy efficiency, limiting the battery’s ability to store and deliver power effectively. You may observe that an aging battery requires more frequent recharging, which disrupts operational efficiency, especially in industrial applications.
Research highlights the importance of analyzing degradation modes to predict battery lifespan accurately. Traditional methods that focus solely on capacity fade and power fade often fail to provide a complete picture. Instead, integrating multiple degradation mechanisms offers a more comprehensive understanding of battery performance over time.
Key findings from degradation studies include:
The necessity of analyzing multiple degradation modes for accurate lifetime prediction.
The inadequacy of traditional methods that only consider capacity and power fade.
The importance of comprehensive modeling to improve battery performance predictions.
Resistance Type | Description of Change | Implication on Capacity Degradation |
---|---|---|
R0 | Minimal increase | Indicates stable performance |
R1 | Accelerated rise | Suggests increase in SEI layer thickness |
R2 | Highest increase | Correlates with significant capacity fade |
Understanding these trends allows you to implement better maintenance practices, ensuring that lithium battery life is extended while maintaining energy efficiency.
3.2 Increased Internal Resistance and Power Decline
Aging lithium-ion batteries experience a rise in internal resistance, which leads to power decline. This increase in resistance occurs due to changes in the battery’s internal components, such as the growth of the SEI layer and the degradation of electrode materials. As a result, the battery’s ability to deliver power efficiently diminishes, affecting its overall performance.
To measure internal resistance, researchers use advanced techniques that provide valuable insights into battery aging:
Measurement Technique | Description |
---|---|
Direct Current (DC) Methods | Includes pulse power tests and Hybrid Pulse Power Characterization (HPPC) tests. |
Alternating Current (AC) Methods | Utilizes electrochemical impedance spectroscopy (EIS) and pulse-multisine measurements. |
Influence of Timescales | Resistance measurements vary significantly based on the timescales of the techniques employed. |
By monitoring internal resistance, you can identify early signs of power fade and take corrective actions to optimize battery performance. Regular maintenance and the use of advanced diagnostic tools are essential for mitigating the effects of increased resistance.
3.3 Safety Risks: Overheating and Thermal Runaway
Aging lithium-ion batteries pose significant safety risks, including overheating and thermal runaway. These risks arise from the chemical and physical changes that occur within the battery over time. For instance, the breakdown of the electrolyte and the growth of the SEI layer can generate excessive heat during operation. In extreme cases, this heat can trigger thermal runaway, leading to a risk of fire or explosion.
Empirical studies provide valuable insights into the thermal safety of lithium-ion batteries:
Research on large-format batteries highlights the thermal runaway characteristics under varying heating conditions.
An electrochemical-thermal coupled model predicts thermal runaway events, offering a statistical framework for assessing overheating risks.
Studies on shallow over-discharge scenarios contribute to understanding the thermal safety of aging batteries.
Experimental investigations assess how different heating methods influence thermal runaway behavior.
The study titled “Safety assessment of Mn-based lithium-ion battery“ discusses how battery size and capacity influence thermal runaway risks. It emphasizes the importance of thermal management systems in preventing overheating and ensuring safety.
To minimize these risks, you should implement robust thermal management systems and adhere to best practices for charging and discharging. These measures not only enhance safety but also extend lithium battery life.
3.4 Implications for Industrial Lithium-Ion Battery Packs
In industrial applications, the aging of lithium-ion battery packs has far-reaching implications. Capacity fade and power fade can disrupt operations, leading to increased downtime and higher maintenance costs. Moreover, the safety risks associated with aging batteries, such as overheating and thermal runaway, pose significant challenges for industrial infrastructure.
A comprehensive analysis of industrial battery packs reveals the following insights:
Aspect | Details |
---|---|
Study Focus | Aging and capacity degradation of lithium-ion battery packs |
Methodology | Experimental data and predictive models used for analysis |
Key Findings | Probabilistic prediction of capacity degradation and inconsistency evolution in aging process |
Time Efficiency | More than 85% time saved in aging experiments compared to conventional methods |
Battery Specifications | LiFePO4 (LFP) cathode, nominal capacity of 100 Ah, aging at different temperatures and current rates |
The degradation rate of lithium-ion batteries in their second life depends on the root cause of degradation during their first life. Batteries with similar first-life state of health (SoH) and degradation causes exhibit comparable degradation rates in their second life. This highlights the importance of understanding the underlying causes of degradation for industrial applications.
To address these challenges, you should invest in advanced battery management systems and predictive maintenance strategies. These solutions help optimize battery performance, reduce downtime, and ensure the safety of industrial lithium-ion battery packs. For customized solutions tailored to your industrial needs, consider consulting experts at Large Power.
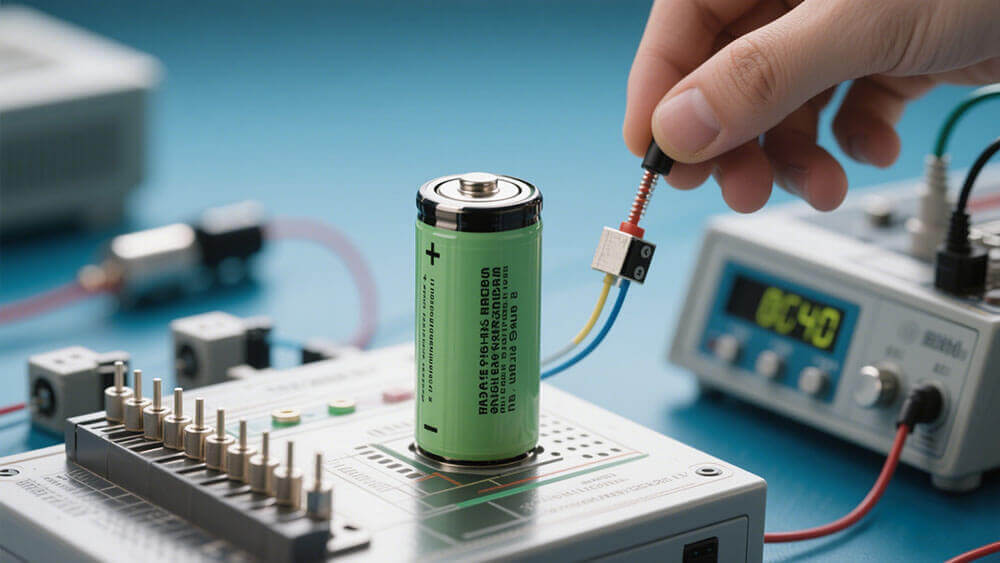
Part 4: Strategies to Mitigate the Gradual Degradation of Lithium Battery
4.1 Best Practices for Charging and Discharging
Adopting proper charging and discharging practices significantly extends the lifespan of lithium-ion batteries. You should maintain the state of charge (SOC) between 20% and 80% to reduce stress on battery chemistry. Avoid overnight charging, as prolonged exposure to full charge accelerates degradation. Recharge before the battery drops below 20% to preserve cell integrity.
Additional tips for optimal charging and discharging:
Avoid charging to 100% whenever possible.
Reduce screen brightness and close unnecessary applications to conserve power.
Implement load leveling by occasionally giving devices a break.
These practices align with research findings, which highlight the importance of cycling frequency and depth of discharge (DoD) in maintaining battery health.
4.2 Temperature Control for Lithium-Ion Batteries
Temperature fluctuations are a leading cause of battery degradation. Maintaining an optimal temperature range of 15-35°C minimizes aging rates. High temperatures accelerate electrolyte breakdown, while low temperatures increase lithium plating.
Finding | Description |
---|---|
Aging Rate Influence | Temperature and discharging rates significantly affect battery aging. |
Optimal Temperature Range | 15-35°C is ideal for extending battery life. |
Degradation Acceleration | Extreme temperatures increase degradation rates. |
You can implement thermal management systems to regulate temperature and prevent overheating. This approach ensures consistent performance and safety.
4.3 Regular Monitoring and Maintenance
Regular monitoring of key indicators like capacity loss and internal resistance helps you identify early signs of degradation. Advanced diagnostic tools, such as electrochemical impedance spectroscopy (EIS), provide precise measurements of battery health. Routine maintenance, including cleaning terminals and inspecting connections, further enhances reliability.
4.4 Advanced Battery Management Systems (BMS)
Modern BMS technology plays a critical role in mitigating battery degradation. These systems monitor voltage, temperature, and state of charge in real time. Features like open-circuit detection and cell balancing algorithms ensure operational safety and efficiency.
Feature | Description |
---|---|
SOC Algorithm | Provides precise state-of-charge estimation. |
SOH Algorithm | Tracks capacity loss and internal resistance for accurate health assessment. |
Real-Time Monitoring | Captures critical data to prevent failures. |
Investing in advanced BMS solutions ensures optimal performance and extends the service life of lithium-ion batteries. For tailored solutions, consult Large Power.
Understanding the causes and effects of lithium-ion battery degradation is essential for maintaining performance and safety. Aging reduces capacity, increases resistance, and raises safety risks like overheating. Proactive measures, such as optimized charging practices and temperature control, help mitigate these issues and extend battery life.
Recycling advancements reduce ecological impacts by 58%.
Proper disposal prevents hazardous waste and environmental contamination.
Lifecycle management improves sustainability and safety.
By addressing degradation, you ensure reliable energy storage and minimize environmental risks.
FAQ
1. How can you tell if a lithium-ion battery is aging?
You can identify aging through signs like reduced capacity, increased charging time, overheating, or voltage fluctuations during operation. Regular monitoring tools can confirm these issues.
2. Does fast charging accelerate battery aging?
Yes, fast charging increases heat and stress on the battery, which accelerates degradation. Use moderate charging rates to extend the battery’s lifespan and maintain safety.
3. What is the safest way to store lithium-ion batteries?
Store batteries in a cool, dry place at 40-60% charge. Avoid extreme temperatures and direct sunlight to minimize degradation and ensure long-term safety.