Contents
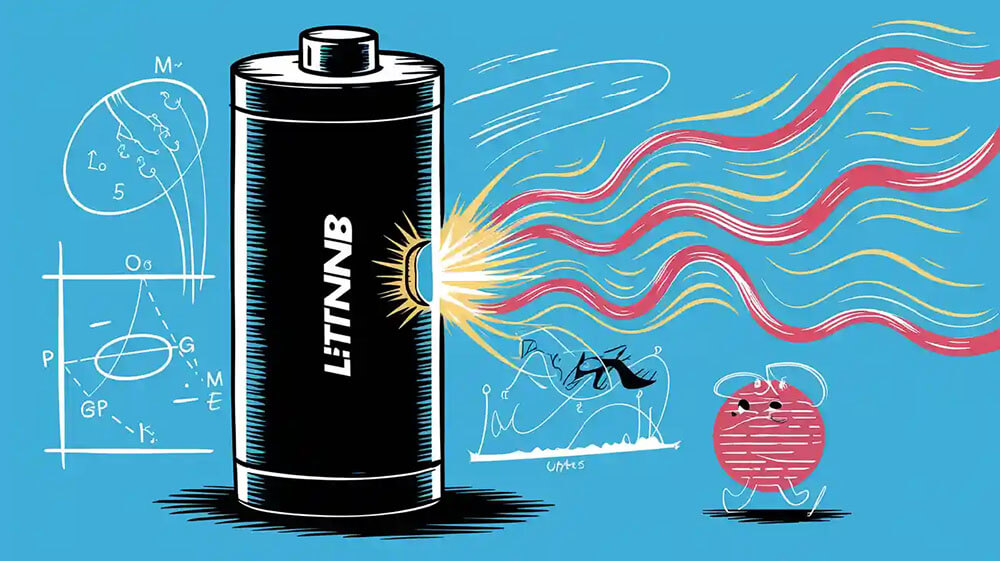
The impact of magnetic fields on lithium-ion batteries is significant, as these fields influence their performance and longevity. They affect the electrochemical reactions occurring within the batteries, which in turn alters their ionic conductivity and thermal stability. You may observe these impacts in various industries that depend on battery technologies, including electric vehicles, renewable energy storage systems, and advanced electronics.
Key Takeaways
Magnetic fields help lithium-ion batteries work better by boosting ion flow and lowering resistance, making energy storage more effective.
Learning how magnetic fields affect battery chemistry can help create safer and longer-lasting batteries for many uses.
Solutions like improving battery design and adding magnetic shields can stop batteries from losing performance in strong magnetic fields.
Part 1: The Science Behind the Impact of Magnetic Fields on Lithium-Ion Batteries
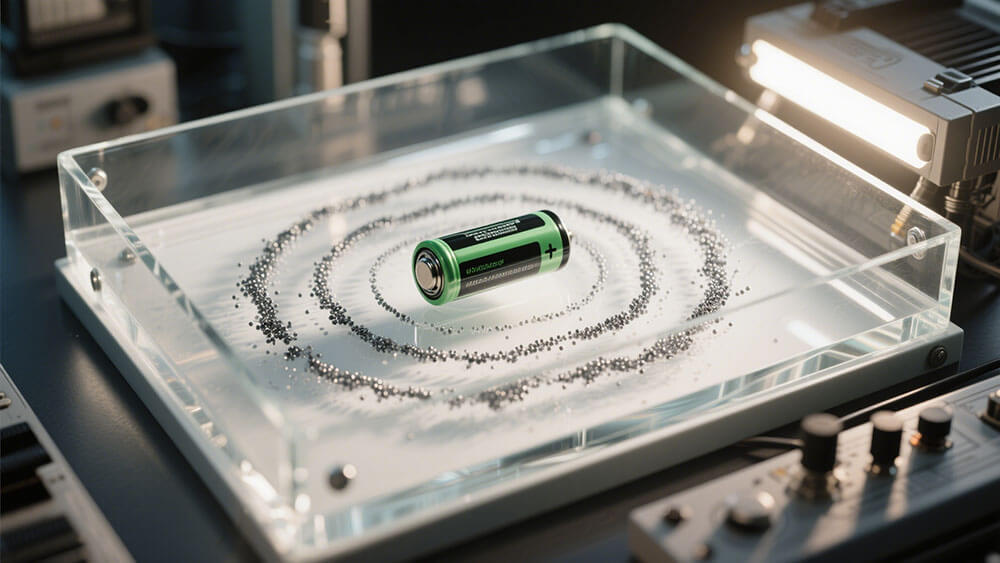
1.1 What Are Magnetic Fields and Their Properties?
Magnetic fields are invisible forces generated by moving electric charges. These fields influence the behavior of charged particles, such as electrons and ions, within their range. You can think of a magnetic field as a region where magnetic forces act on objects with magnetic properties.
Magnetic fields have several key properties:
Dipole Nature: Magnetic fields originate from a pair of poles, north and south, which create a dipole structure.
Magnetic Field Intensity: This measures the strength of the field and is represented as a vector quantity.
Permeability and Susceptibility: These properties describe how materials respond to magnetic fields. Permeability measures the ability of a material to support the formation of a magnetic field, while susceptibility indicates how easily a material becomes magnetized.
Measurement Units: The strength of a magnetic field is measured in teslas (T), with one tesla representing one newton per ampere-meter.
Magnetic fields play a crucial role in various industries, including robotics, medical devices, and infrastructure systems. For example, in robotics, magnetic sensors help guide autonomous machines.
1.2 Interaction Between Magnetic Fields and Battery Electrochemistry
Magnetic fields can significantly influence the electrochemical processes within lithium-ion batteries. These effects are particularly evident in the movement of lithium ions and the overall battery performance.
When a magnetic field interacts with a lithium-ion battery, it alters the behavior of charged particles. This interaction can enhance ionic conductivity, reduce polarization, and improve charge transfer kinetics. For example, studies have shown that the presence of a magnetic field decreases charge transfer resistance, leading to faster lithium-ion diffusion.
Evidence Type | Findings | Description |
---|---|---|
Charge Transfer Resistance | Improved | Magnetic fields reduce resistance, enhancing ion kinetics. |
Diffusion Resistance | Reduced | Better mass transport and lower interfacial resistance. |
Capacity Retention | Fluctuates | Initial improvement, but decreases when the field is removed. |
Additionally, magnetic fields can suppress the growth of lithium dendrites, which are a major cause of short circuits and battery failure. Advanced diagnostic tools, such as electromagnetic imaging, have been used to study these effects in real-time.
Mechanism | Description |
---|---|
Magnetic Field Suppression | Prevents lithium dendrite growth. |
Electromagnetic Diagnostics | Enables real-time monitoring of lithium plating. |
Understanding the interaction between magnetic fields and battery electrochemistry is essential for optimizing the design of electrochemical energy storage systems. This knowledge can lead to safer and more efficient batteries for applications in medical devices, security systems, and industrial equipment.
Part 2: Effects of Magnetic Fields on Lithium-Ion Battery Performance
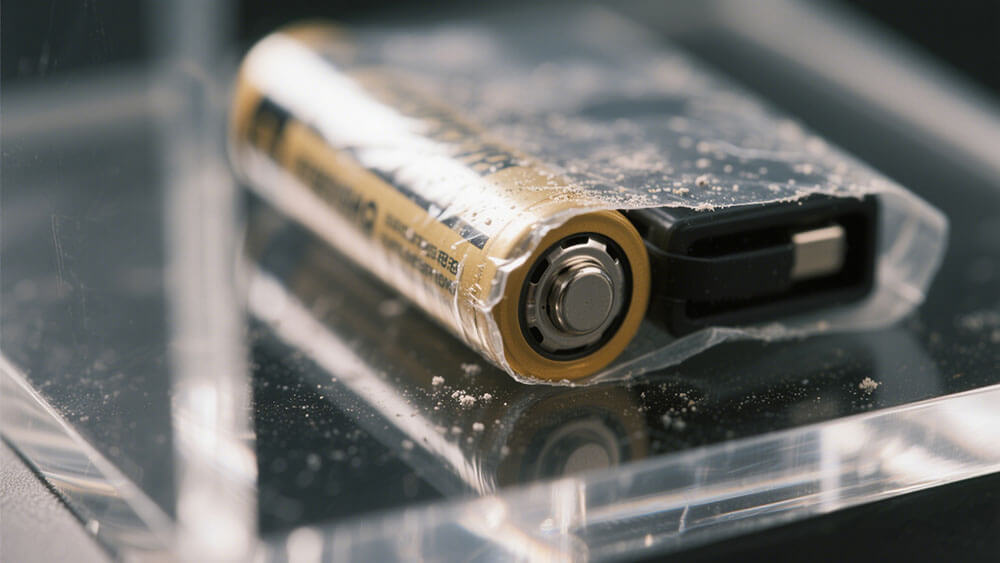
2.1 Enhancing Ionic Conductivity and Reducing Polarization
Magnetic fields play a pivotal role in improving the ionic conductivity of lithium-ion batteries. By influencing the movement of lithium ions within the electrolyte, magnetic fields enhance the efficiency of ion transport. This improvement directly reduces polarization, a phenomenon that occurs when the flow of ions is hindered, leading to uneven reaction distribution across the electrodes.
Polarization often results from low porosity in composite electrodes, which restricts ionic conductivity. Research highlights that enhancing ionic conductivity mitigates this issue, ensuring better mass transport dynamics and uniform reaction distribution. The table below summarizes these findings:
Finding | Description |
---|---|
Low Porosity | Composite electrodes with low porosity lead to large polarization during high-rate discharge reactions. |
Ionic Conductivity | Low porosity correlates with low effective ionic conductivity, affecting reaction distribution. |
Performance Impact | Enhancing ionic conductivity is crucial for improving battery performance and reducing polarization. |
For industries like robotics, where precision and reliability are paramount, reducing polarization ensures consistent power delivery. Similarly, in medical devices, enhanced ionic conductivity supports the stable operation of life-critical equipment.
2.2 Influence on Thermal Stability and Safety
Thermal stability is a critical factor in the performance and safety of lithium-ion batteries. Magnetic fields influence the thermal behavior of these batteries by altering the heat generation and dissipation processes during charge and discharge cycles. By improving ionic conductivity and reducing internal resistance, magnetic fields minimize heat buildup, thereby enhancing thermal stability.
Moreover, magnetic fields can suppress the formation of lithium dendrites, which are a major cause of short circuits and thermal runaway. This suppression not only improves safety but also extends the lifespan of the battery. Advanced diagnostic tools, such as electromagnetic imaging, have been instrumental in studying these effects, providing valuable insights for designing safer battery systems.
2.3 Long-Term Impacts on Battery Degradation and Lifespan
The long-term impact of magnetic fields on lithium-ion batteries extends beyond immediate performance improvements. By enhancing ionic conductivity and reducing polarization, magnetic fields contribute to slower degradation of battery components. This effect is particularly significant for applications requiring prolonged operational life, such as consumer electronics and industrial systems.
For instance, in consumer electronics like smartphones and laptops, reduced degradation translates to longer battery life and fewer replacements. Explore more about consumer electronics applications. In industrial settings, where downtime can lead to significant losses, extended battery lifespan ensures uninterrupted operations and cost savings.
However, the removal of magnetic fields can sometimes lead to a decline in capacity retention, as observed in certain studies. This highlights the need for consistent magnetic field application or alternative strategies to maintain the benefits. Future research aims to address these challenges, paving the way for innovative solutions that optimize the impact of magnetic fields on lithium-ion batteries.
Part 3: Practical Implications for Lithium-Ion Battery Systems in Industry
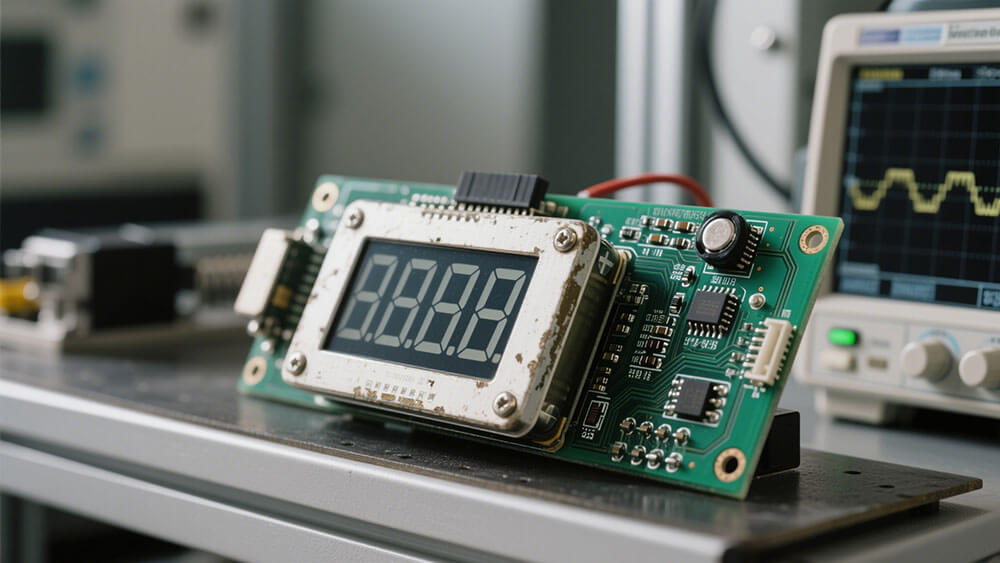
3.1 Challenges in High-Magnetic-Field Environments
High-magnetic-field environments pose significant challenges for lithium-ion batteries. These fields can disrupt the electrochemical processes within the battery, leading to performance degradation and safety risks. For instance, research from the MagLab highlights the formation of dendrites in solid-state lithium batteries, which can cause short circuits and eventual failure. This issue becomes more pronounced in high-energy-density materials like silicon and lithium metal, where electrode expansion during cycling leads to mechanical deformation and cell failure.
Industries such as robotics and medical devices often operate in environments with fluctuating magnetic fields. These conditions can exacerbate battery degradation, reducing operational efficiency and increasing maintenance costs. Understanding these challenges is critical for designing robust battery systems that can withstand such demanding conditions.
3.2 Mitigation Strategies for Magnetic Field Interference
To address the challenges posed by magnetic fields, industries have developed several mitigation strategies. One effective approach involves optimizing the battery’s internal structure to minimize the impact of magnetic interference. For example, using advanced separators and electrolytes can reduce the risk of dendrite formation and improve thermal stability.
Another strategy focuses on external shielding. By incorporating magnetic shielding materials into the battery housing, you can protect the internal components from external magnetic fields. Case studies reveal that improving capacity efficiency in batteries used in high-magnetic-field environments could save industries up to $970,800 annually. These savings highlight the importance of adopting effective mitigation techniques
Magnetic fields significantly affect lithium-ion batteries by altering their efficiency, safety, and lifespan. You must address these effects to ensure optimal battery performance. Mitigation strategies, such as shielding and structural optimization, can help you maintain reliability. Continued research will unlock new ways to harness magnetic fields, enhancing the power and potential of lithium-ion batteries across industries.
FAQ
1. What is the impact of magnetic fields on lithium-ion batteries?
Magnetic fields influence electrochemical reactions, mass transport dynamics, and thermal stability, improving performance but posing challenges in high-magnetic-field environments.
2. How do magnetic field effects on electrochemical reactions improve battery performance?
Magnetic fields enhance ionic conductivity and reduce polarization, optimizing mass transport dynamics and improving the efficiency of electrochemical energy storage system.
3. Can magnetic field effects on mass transport extend battery lifespan?
Yes, magnetic fields improve ion movement, reducing degradation and extending the lifespan of lithium-ion batteries in demanding applications.
Tip: For professional guidance on battery lifespan, visit Large Power.