Contents
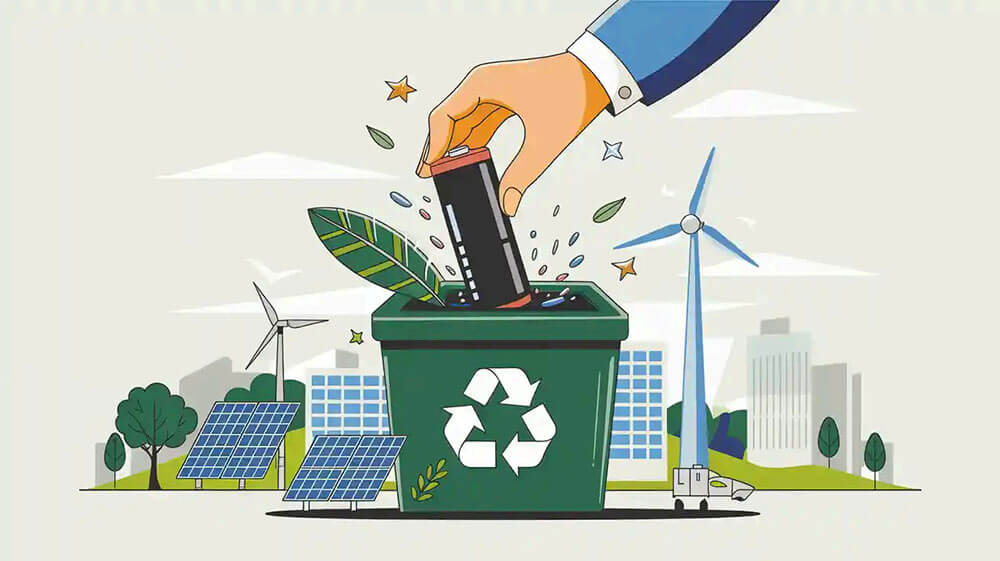
Lithium-ion batteries play a pivotal role in powering industries like medical devices, robotics, and consumer electronics. For instance, in 2023, over 31% of the lithium-ion battery market was driven by consumer electronics, while medical applications saw rising demand due to technological advancements and aging populations. Improper disposal of batteries causes secondary pollution about lithium battery waste, impacting ecosystems and industrial sustainability. Recycling these batteries reduces environmental impacts by up to 58%, recovering valuable materials while minimizing pollution.
Key Takeaways
Recycling lithium-ion batteries helps the environment and saves materials.
Throwing batteries away can pollute soil, water, and air. This is dangerous for health.
Using better recycling methods, like hydrometallurgy, can save money and recover more.
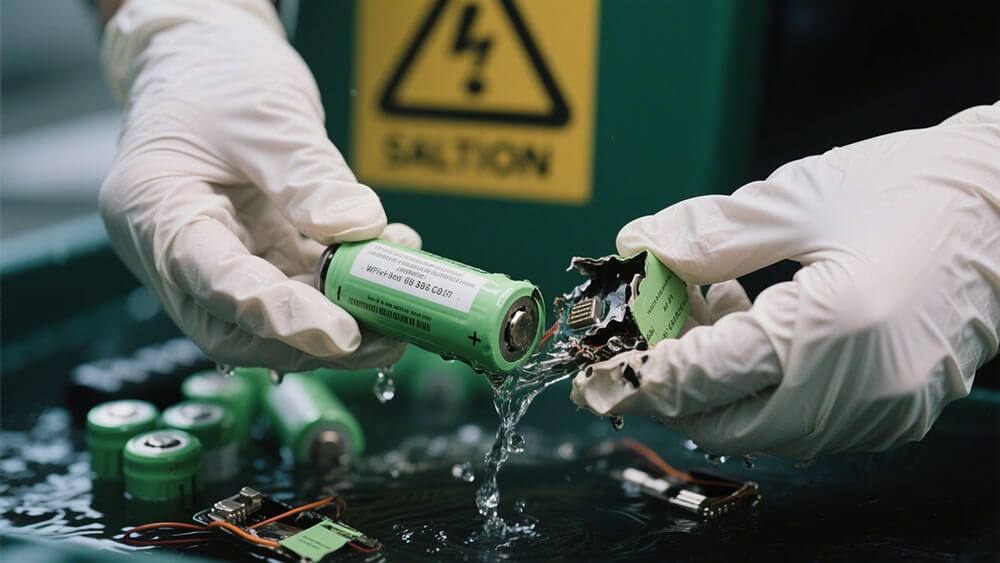
Part 1: Understanding Secondary Pollution About Lithium Battery Disposal
1.1 What is Secondary Pollution in Lithium-Ion Battery Recycling?
Secondary pollution about lithium battery disposal refers to the indirect environmental damage caused by improper handling of used batteries during or after their recycling process. When lithium-ion batteries are discarded without proper protocols, they can release harmful chemicals and heavy metals into the environment. These pollutants often infiltrate soil, water, and air, creating long-term ecological and health risks.
For example, corroded batteries can leak toxic substances like nickel, cadmium, and cobalt into the soil. These chemicals contaminate groundwater and surface water, disrupting aquatic ecosystems and rendering water sources unsafe for consumption. Additionally, landfill fires caused by improperly disposed batteries release hazardous gases into the atmosphere, contributing to air pollution and global warming. Such fires are not uncommon; between June 2017 and December 2020, 124 fires were reported in a landfill in the Pacific Northwest due to lithium-ion batteries. This trend highlights the growing risks of secondary pollution.
1.2 Environmental Risks of Improper Disposal
Improper disposal of lithium-ion batteries poses significant environmental concerns. Chemicals from burning batteries can vaporize, leading to air contamination that contributes to acid rain and further pollutes water sources. Moreover, leaked metals such as nickel and cadmium are classified as carcinogens, posing severe health risks to humans and animals.
A study from Australia revealed that 98.3% of lithium-ion batteries end up in landfills, where they risk contaminating soil, water, and air. This widespread disposal practice exacerbates environmental degradation. In addition to pollution, landfill fires caused by these batteries have increased over the years, with reported incidents rising from 21 in 2018 to 47 in 2020. These fires not only release toxic fumes but also pose secondary fire hazards, endangering nearby communities and ecosystems.
1.3 Impacts on Industrial and Infrastructure Sectors
The improper disposal of lithium-ion batteries also affects industrial and infrastructure sectors. Recycling these batteries can significantly reduce CO₂ emissions—up to 40% compared to mining raw materials. This reduction supports sustainability goals and mitigates the environmental footprint of industries reliant on lithium-ion batteries, such as automotive and robotics.
The automotive sector alone contributes over 80% of global battery waste generation, underscoring the need for efficient recycling processes. Additionally, the global secondary use market for recycled lithium-ion batteries is projected to exceed $5 billion by 2030, offering economic incentives for industries to adopt sustainable practices. Recycled lithium is estimated to be 30% cheaper than newly mined lithium, making it a cost-effective solution for manufacturers. Furthermore, the recycling industry is expected to create over 50,000 new jobs globally by 2030, driving economic growth while addressing environmental concerns.
Impact Description | Measurable Effect |
---|---|
CO₂ Emissions Reduction | Up to 40% reduction compared to raw material mining |
Automotive Sector Contribution | Over 80% of global battery waste generation |
Global Secondary Use Market | Projected to exceed $5 billion by 2030 |
Cost of Recycled Lithium | Estimated to be 30% cheaper than newly mined lithium |
Job Creation | Expected to create over 50,000 new jobs globally by 2030 |
By addressing secondary pollution about lithium battery disposal, industries can enhance sustainability while reducing costs and environmental impacts. For more insights into sustainable practices, visit Sustainability at Large Power.
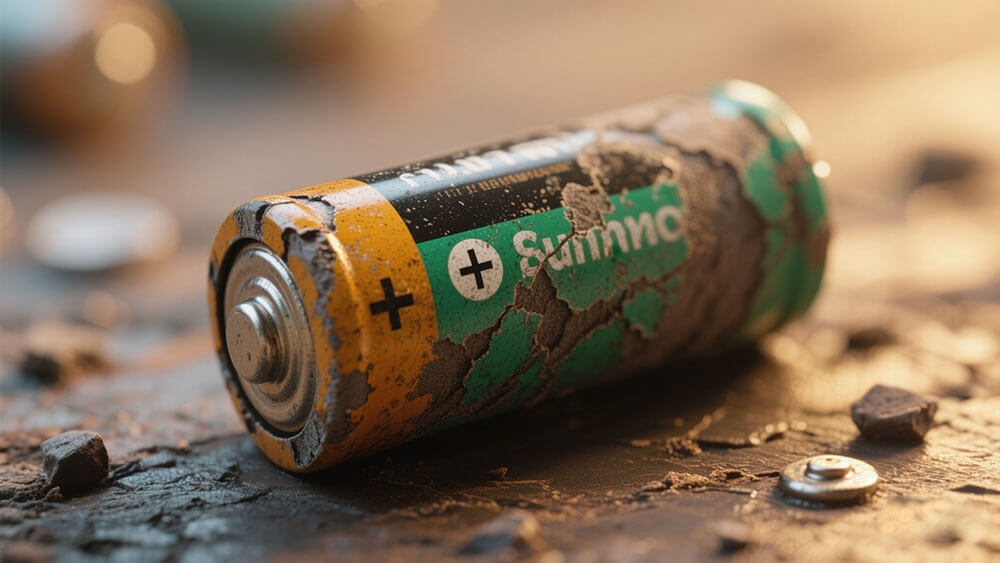
Part 2: Challenges in the Recycling Process for Lithium-Ion Batteries
2.1 Technical Challenges: Complex Battery Chemistry and Design
Recycling lithium-ion batteries presents significant technical hurdles due to their intricate chemistry and design. For example, NMC Lithium batteries, widely used in robotics and consumer electronics, require advanced processes like hydrometallurgy and pyrometallurgy to recover valuable materials. These methods demand a deep understanding of multiphase flow phenomena to optimize recovery rates and selectivity. The complexity of battery chemistry directly impacts the efficiency of recycling efforts, making it essential for industries to invest in specialized technologies. Without addressing these challenges, the recycling process risks inefficiencies that could undermine sustainability goals.
2.2 Logistical Challenges: Collection, Sorting, and Transportation
The logistics of recycling lithium-ion batteries involve multiple layers of complexity. You must navigate stringent regulations like the EU Battery Regulation, which introduces sustainability targets and carbon footprint declarations. These requirements complicate the collection and transportation of batteries, especially in regions with underdeveloped recycling infrastructure, such as Italy. Additionally, sorting batteries into critical and non-critical categories requires specialized handling. Critical batteries demand immediate quarantine and expert management, while non-critical batteries are stored and transported to sorting facilities. Producer Responsibility Organizations (PROs) oversee compliance with collection targets, adding another layer of logistical challenges. These factors highlight the need for streamlined processes to ensure efficient recycling.
2.3 Economic Challenges: Balancing Costs and Sustainability
Balancing costs and sustainability remains a key challenge in the recycling process. Hydrometallurgical recycling offers cost savings of 11.3% compared to virgin materials, with a processing cost of $24.64 per kilogram of cell. However, pyrometallurgical methods, costing $29.10 per kilogram, are less cost-effective. Industries must weigh these options carefully to achieve both economic and environmental benefits. The cost of virgin materials, at $27.74 per kilogram, further underscores the importance of adopting sustainable recycling methods. By prioritizing cost-effective solutions, you can reduce expenses while contributing to a circular economy for lithium-ion batteries.
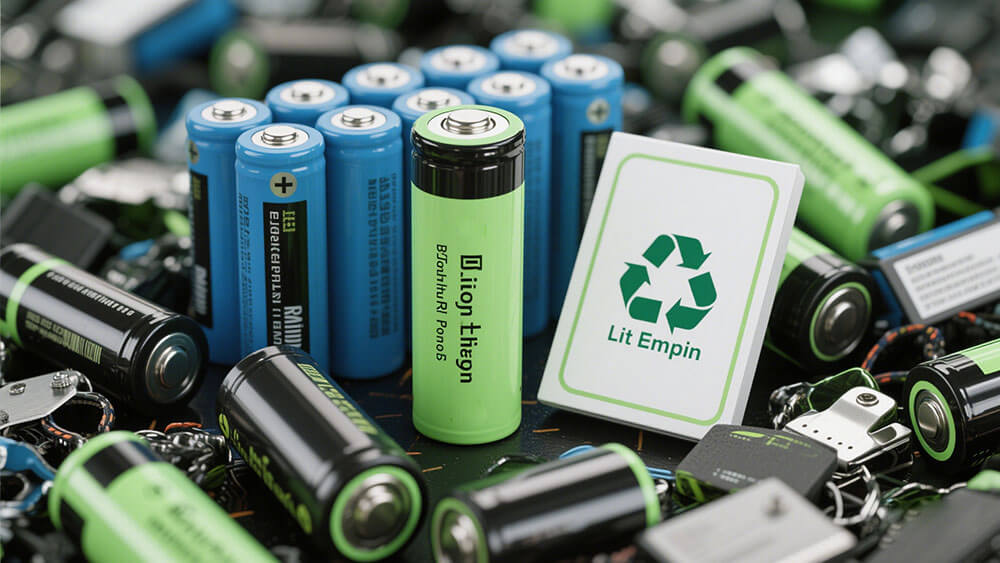
Part 3: Effective Recycling Methods to Recycle Batteries
3.1 Mechanical Recycling: Overview and Benefits
Mechanical recycling is one of the most straightforward methods to recycle batteries. This process involves the physical separation of battery components, such as metals, plastics, and electrolytes, without altering their chemical structure. You can achieve this through shredding, crushing, and sieving techniques.
The simplicity of mechanical recycling offers several advantages:
Lower environmental impact: Compared to hydrometallurgical and pyrometallurgical methods, mechanical recycling generates fewer emissions and consumes less energy.
Cost-effectiveness: The process requires minimal chemical inputs, reducing operational costs.
Scalability: Mechanical recycling can be easily scaled to handle large volumes of lithium-ion batteries.
However, this method has limitations. It typically results in lower recovery rates for valuable metals like cobalt and nickel, which impacts its economic viability. Recent studies emphasize the need for advanced techniques to improve material recovery rates.
3.2 Pyrometallurgical Recycling: Process and Limitations
Pyrometallurgical recycling involves high-temperature processes to extract metals from lithium-ion batteries. This method uses furnaces to smelt battery components, recovering materials like cobalt, nickel, and copper. The process is highly efficient for certain metals, with recovery rates reaching up to 98% for cobalt and nickel.
Material | Recovery Efficiency | Notes |
---|---|---|
Cobalt | 98% | High recovery efficiency, crucial for economic viability. |
Lithium | 90% | Requires additional processing, increasing costs. |
Nickel | 98% | Efficient recovery similar to cobalt. |
Copper | 90% | Efficient recovery across all processes. |
Aluminum | 90% | Lost in slag, requires further processing for recovery. |
Despite its efficiency, pyrometallurgical recycling has notable drawbacks. The high energy consumption and greenhouse gas emissions raise environmental concerns. Additionally, some materials, such as aluminum and lithium, are lost in the slag and require further processing. These limitations highlight the need for more sustainable alternatives.
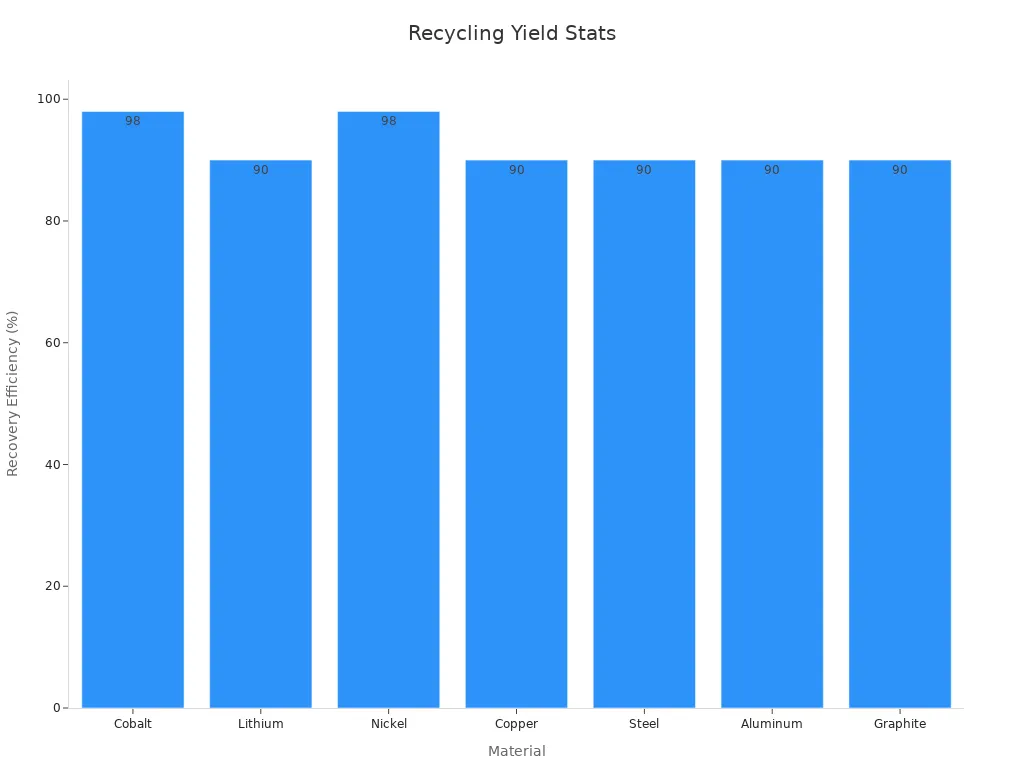
3.3 Hydrometallurgical Recycling: Efficiency and Environmental Impact
Hydrometallurgical recycling uses chemical solutions to dissolve and recover metals from lithium-ion batteries. This method is highly efficient, with recovery rates exceeding 90% for critical materials like lithium, cobalt, and nickel. It also offers a lower environmental footprint compared to pyrometallurgical processes.
Key environmental benefits of hydrometallurgical recycling include:
Recycling lithium-ion batteries emits less than half the greenhouse gases compared to conventional mining.
It uses about one-fourth of the water and energy required for mining new metals.
For the scrap stream, the recycling process results in:
19% of the GHG emissions of mining and processing.
12% of the water use.
11% of the energy use.
The Environmental Efficiency Index (EEI) and Technical Efficiency Index (TEI) are valuable metrics for assessing the sustainability and performance of hydrometallurgical recycling. These indices consider factors like energy consumption, greenhouse gas emissions, and material recovery rates.
Note: While hydrometallurgical recycling is effective, it requires careful management of chemical waste to prevent secondary pollution.
3.4 Emerging Techniques: Direct Recycling and Biometallurgical Approaches
Emerging recycling technologies aim to address the limitations of traditional methods. Direct recycling and biometallurgical approaches are two promising innovations in this field.
Direct Recycling: This method focuses on recovering and reconditioning battery components, such as cathodes and electrolytes, without breaking them down into raw materials. Direct recycling preserves the structural integrity of materials, reducing energy consumption and costs. It is particularly effective for NMC Lithium batteries used in robotics and consumer electronics.
Biometallurgical Approaches: These techniques use microorganisms to extract metals from batteries. Biometallurgy offers a low-energy, environmentally friendly alternative to conventional methods. It is still in the experimental stage but shows great potential for scalability.
Both methods align with sustainability goals by minimizing waste and reducing the environmental impact of the recycling process. As these technologies mature, they could revolutionize the way you recycle batteries.
Trip:To explore custom battery solutions tailored to your needs, visit Custom Battery Solutions.
Proper recycling of lithium-ion batteries is essential to prevent secondary pollution and protect the environment. Businesses play a critical role in advancing sustainable practices by investing in innovative recycling technologies. For example, direct recycling reduces energy consumption by 15% and costs by 50%, making it an efficient solution for industries reliant on batteries.
Collaboration among industries, governments, and recycling facilities drives the creation of a circular economy. Multisector partnerships, regulatory frameworks, and reverse logistics systems ensure efficient battery collection and recycling. These initiatives promote sustainability while addressing technical challenges in the recycling process.
You can explore tailored solutions to meet your specific needs by visiting Custom Battery Solutions.
FAQ
1. What are the main benefits of recycling lithium-ion batteries?
Recycling lithium-ion batteries reduces environmental harm, recovers valuable materials, and supports sustainability goals. It also lowers costs for industries reliant on batteries, such as robotics and infrastructure.
2. How can businesses ensure efficient recycling processes?
You can partner with certified recycling facilities, implement reverse logistics systems, and invest in advanced technologies like hydrometallurgical methods to optimize material recovery and minimize secondary pollution.
3. Why choose Large Power for custom battery solutions?
Large Power offers tailored solutions for industrial, medical, and consumer electronics applications. Explore our expertise and sustainable practices at custom battery solutions.