Contents
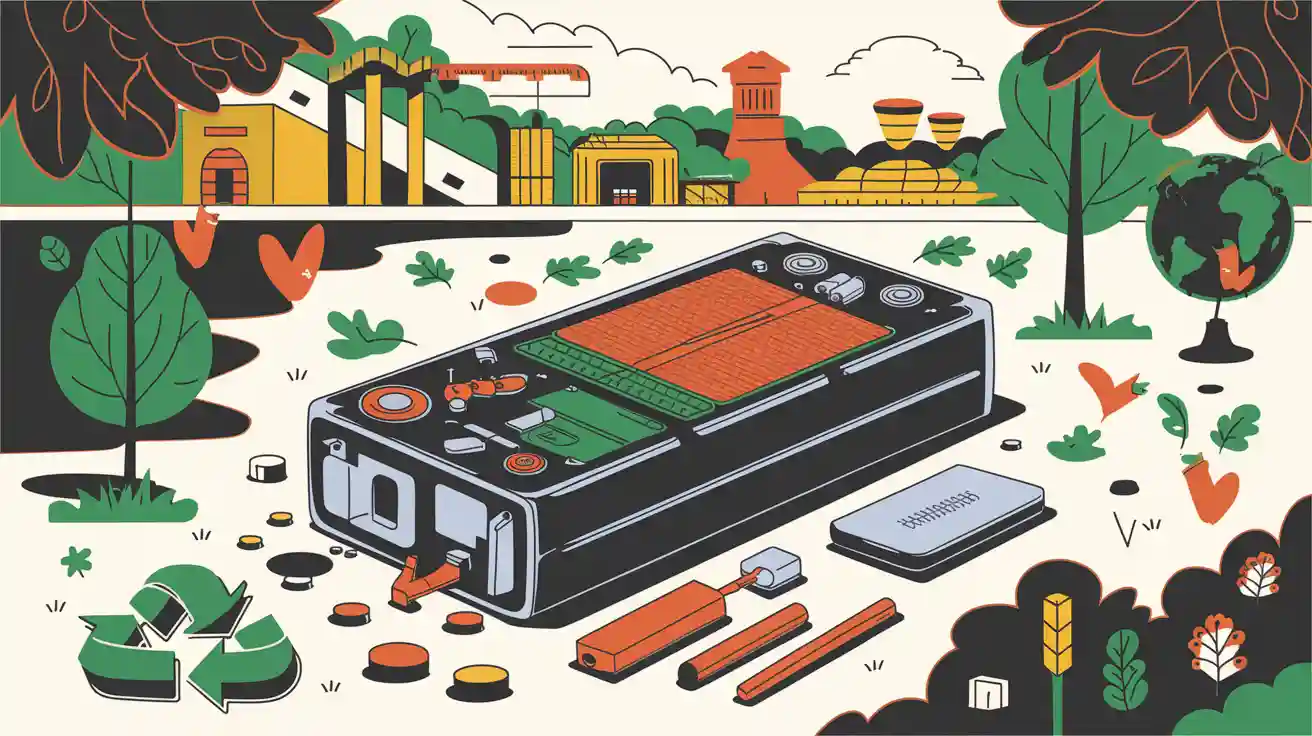
Lithium-ion battery recycling plays a crucial role in reducing environmental harm and conserving resources. As batteries make up 87% of global lithium usage, improper disposal leads to leaching of harmful chemicals into soil and water. Recycling not only mitigates these risks but also reduces the need for mining, conserving non-renewable materials. With a projected recycling rate of 30% by 2024, industries must refine the lithium-ion battery scrapping criterion to maximize material recovery and minimize waste.
Key Takeaways
Recycling lithium-ion batteries helps the planet by saving resources and cutting down on harmful waste. This is key for a better future.
Using clean energy in recycling centers can cut pollution and save energy. This makes recycling even better for the environment.
Setting clear rules for battery disposal and improving recycling methods can recover more materials, create less waste, and help reuse resources.
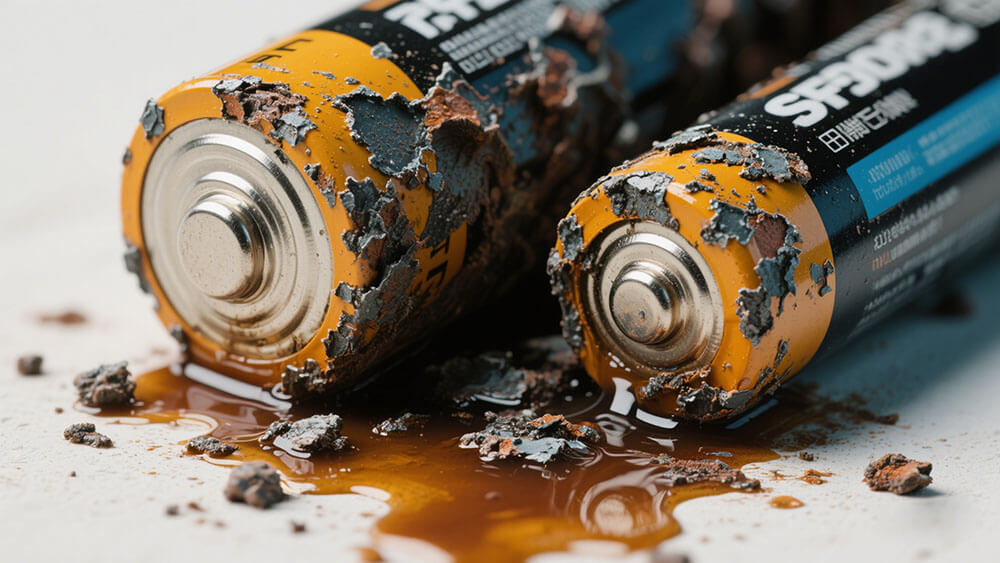
Part 1: Key Environmental Challenges in Lithium-Ion Battery Recycling
1.1 Energy Consumption and Carbon Footprint
Recycling lithium-ion batteries requires significant energy, which contributes to greenhouse gas emissions. The processes involved, such as crushing, sorting, and extracting valuable materials, often rely on energy-intensive machinery. If the energy used comes from non-renewable sources, the carbon footprint of recycling increases. This undermines the environmental benefits of lithium-ion battery recycling.
You can reduce these emissions by adopting renewable energy sources in recycling facilities. Solar, wind, or hydroelectric power can significantly lower the environmental impacts of energy consumption. Additionally, optimizing recycling technologies to improve energy efficiency can further minimize the carbon footprint.
1.2 Toxic Waste and Hazardous Byproducts
Lithium-ion battery recycling generates toxic waste, including heavy metals like cobalt, nickel, and manganese. These substances pose risks to human health and the environment if not handled properly. Improper disposal or inadequate recycling methods can lead to soil and water contamination, harming ecosystems and communities.
To address this challenge, you should ensure that recycling facilities comply with strict environmental regulations. Advanced recycling methods, such as hydrometallurgy, can help reduce hazardous byproducts. This process uses chemical solutions to extract valuable materials, minimizing the release of harmful substances. However, it requires careful management to prevent secondary pollution.
1.3 Lithium-Ion Battery Scrapping Criterion and Material Recovery Inefficiencies
The lithium-ion battery scrapping criterion plays a crucial role in determining the efficiency of material recovery. Inefficiencies in this process lead to the loss of valuable materials and increased waste. Several factors contribute to these inefficiencies:
Factor | Description |
---|---|
Complex Battery Specifications | Early NEV batteries have varied specifications, complicating recycling efforts and preventing cost reduction through scale. |
Presence of Illegal Enterprises | Many illegal operations utilize inadequate recycling technologies, further diminishing recovery efficiency. |
Lack of Uniform Standards | The absence of consistent recycling standards limits the effectiveness of recovery processes across the industry. |
You can improve material recovery by standardizing the lithium-ion battery scrapping criterion. Establishing uniform guidelines for battery design and recycling processes can enhance efficiency. Additionally, investing in advanced technologies and eliminating illegal recycling operations can help maximize material recovery and reduce waste.
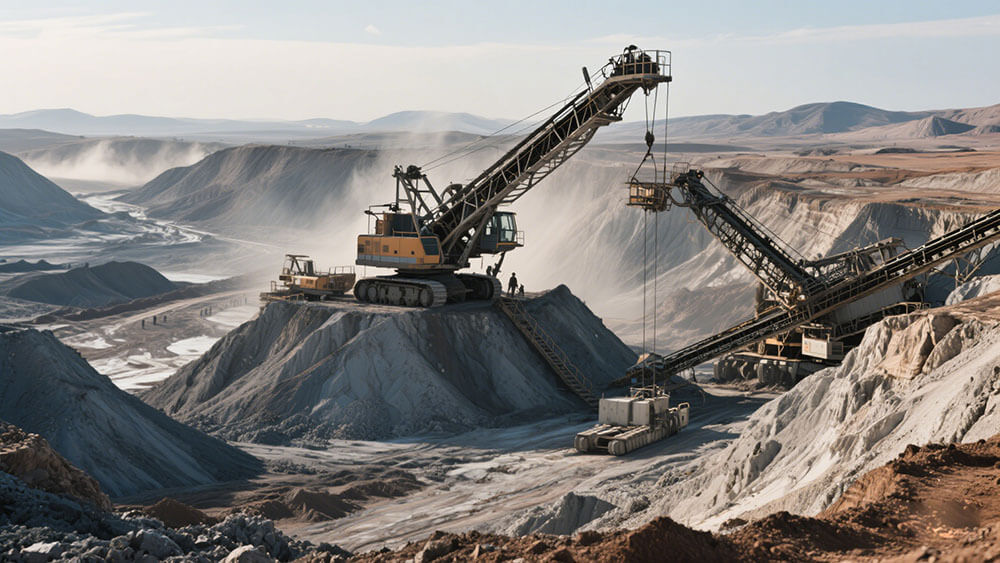
Part 2: Recycling vs. Mining and Second-Life Applications
2.1 Environmental Impacts of Mining for Battery Materials
Mining for battery materials like lithium, cobalt, and nickel significantly impacts the environment. Extracting these resources consumes vast amounts of energy and water while emitting greenhouse gases. For instance:
Mining 1 kg of NCA-equivalent battery-grade material requires 193.9 MJ of energy.
Water consumption for the same amount reaches 77.3 liters.
CO2-equivalent emissions amount to 14.5 kg per kg of material mined.
These figures highlight the substantial environmental footprint of mining. Additionally, mining operations often lead to habitat destruction and the release of toxic chemicals into surrounding ecosystems. This not only threatens biodiversity but also endangers local communities relying on these ecosystems for their livelihoods.
Recycling lithium-ion batteries offers a more sustainable alternative. By recovering valuable materials from used batteries, you can reduce the need for mining and its associated environmental costs. Recycling reduces environmental impacts by up to 99% compared to conventional mining. This shift supports the circular economy by keeping materials in use for longer, minimizing waste, and conserving natural resources.
2.2 Second-Life Applications: Opportunities and Limitations
Second-life applications for lithium-ion batteries present exciting opportunities for extending their usability. After their initial use in electric vehicles or consumer electronics, batteries often retain 70-80% of their original capacity. Repurposing these batteries can provide cost-effective energy storage solutions for various applications. Notable examples include:
Nissan European Headquarters, Paris: A system using 12 second-life Nissan Leaf batteries with a 192 kWh capacity for energy management.
Robert Mondavi Institute, UC Davis: A 300-kWh system integrating solar PV and repurposed Nissan Leaf battery packs.
Johan Cruijff Arena, Amsterdam: A hybrid system with 148 Nissan Leaf batteries, offering 3 MW power capacity and 2.8 MWh storage.
Lunen, Germany: A 13 MWh system utilizing 1,000 BMW i3 packs, with 90% being second-life batteries.
These projects demonstrate the potential of second-life batteries to reduce energy costs, optimize electricity usage, and provide backup power. However, limitations exist. Second-life batteries often have reduced capacity and efficiency compared to new ones. Performance variability among different makes and models complicates their reliability. These factors create unpredictability, limiting their market potential.
Despite these challenges, second-life applications contribute to environmental sustainability by delaying the disposal of batteries and reducing the demand for new materials. They align with the principles of the circular economy, promoting resource efficiency and waste reduction.
2.3 Battery Recycling as a Sustainable Alternative: Environmental Trade-Offs
Battery recycling offers a sustainable alternative to mining, but it comes with its own environmental trade-offs. Recycling processes, such as hydrometallurgy and pyrometallurgy, consume energy and produce emissions. However, the environmental benefits outweigh these costs. Recycling reduces water consumption by 87.7% for scrap and 72.2% for battery streams compared to mining. Greenhouse gas emissions are also 35% lower for circular refinement processes.
A life cycle assessment reveals that recycling lithium-ion batteries reduces environmental impacts by at least 58% compared to mining. This makes recycling a critical component of a sustainable battery supply chain. By adopting advanced recycling technologies and integrating renewable energy into recycling facilities, you can further minimize the environmental footprint of these processes.
Battery recycling not only conserves resources but also prevents the release of toxic chemicals into the environment. It supports the transition to a circular economy, ensuring that valuable materials are reused rather than discarded. As industries increasingly prioritize environmental sustainability, recycling will play a pivotal role in reducing the ecological impact of battery production and disposal.
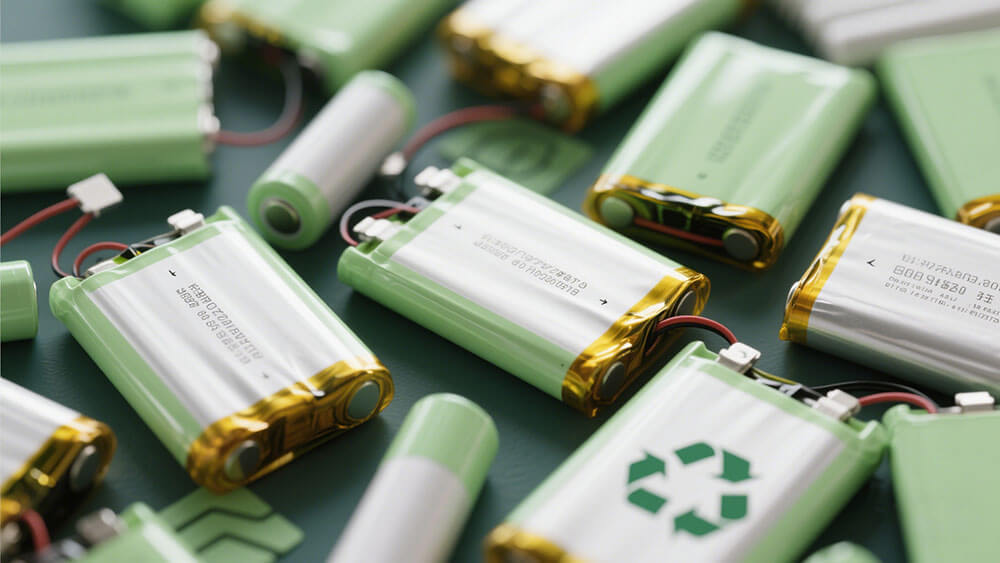
Part 3: Environmental Impacts of Recycling Technologies
3.1 Hydrometallurgy: Efficiency and Environmental Considerations
Hydrometallurgy is a widely used recycling method for lithium-ion batteries. It involves using chemical solutions to extract valuable materials like lithium, cobalt, and nickel. This process achieves high recycling rates and material purity, making it an efficient option for sustainable waste management. For example:
Product | Recycling Rate (%) | Purity (%) | Remarks |
---|---|---|---|
Graphite | >98 | >99.5 | High marketable value for anode reuse |
Cemented Copper | 94-99 | N/A | Valuable for Cu smelting |
Co, Ni, Mn Salts | 95 | N/A | Raw material for new cathode synthesis |
Li2CO3 | 48-64 | 16.8-19.2 | Important for Li industry |
Despite its efficiency, hydrometallurgy has environmental drawbacks. It consumes significant energy and generates wastewater that requires careful treatment. You can minimize these impacts by adopting renewable energy sources and improving wastewater management systems.
3.2 Pyrometallurgy: Challenges in Minimizing Environmental Waste
Pyrometallurgy uses high temperatures to recover metals from retired electric vehicle batteries. This method is less complex than hydrometallurgy but faces challenges in minimizing environmental waste. It produces greenhouse gas emissions and consumes substantial energy. However, compared to mining virgin materials, pyrometallurgy significantly reduces energy consumption and emissions:
Process Type | Energy Consumption (MJ/kg cell) | GHG Emissions (g/kg cell) |
---|---|---|
Pyrometallurgy | 4.59 | 1224.12 |
Virgin Materials | 297.51 | 20847.50 |
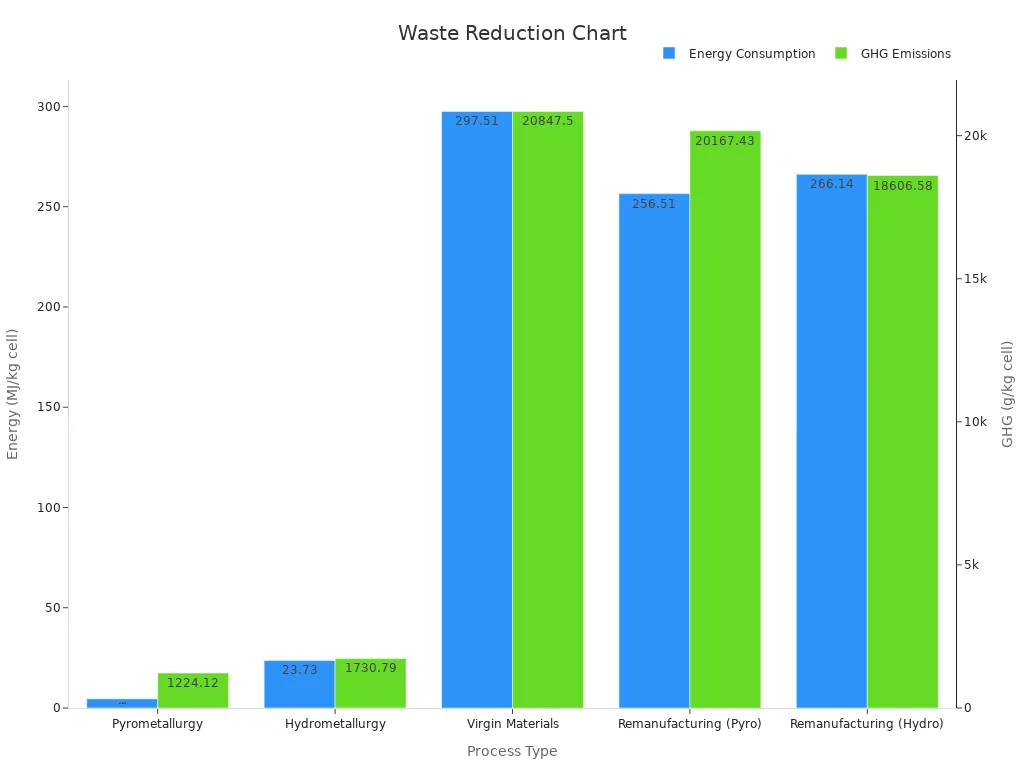
To enhance its environmental benefits, you should explore hybrid recycling processes that combine pyrometallurgy with other methods, reducing waste and improving efficiency.
3.3 Emerging Technologies: Innovations for Reducing Environmental Impacts
Emerging technologies are transforming recycling processes by improving efficiency and reducing environmental impacts. Design for Recycling (DfR) optimizes battery designs to enhance recyclability. Modular designs simplify disassembly, lowering energy consumption during recycling. Life Cycle Assessment (LCA) combined with Structural Equation Modeling (SEM) provides insights into the environmental performance of recycling methods, enabling better decision-making.
Innovative approaches like prefabrication and 3D printing also show promise. These technologies improve recycling rates and support sustainable waste management. Scenario analyses suggest a 27–35% annual growth potential for these methods, highlighting their economic performance and environmental benefits.
By adopting these innovations, you can achieve higher recovery rates for valuable materials like lithium and cobalt, reducing the overall environmental footprint of battery recycling.
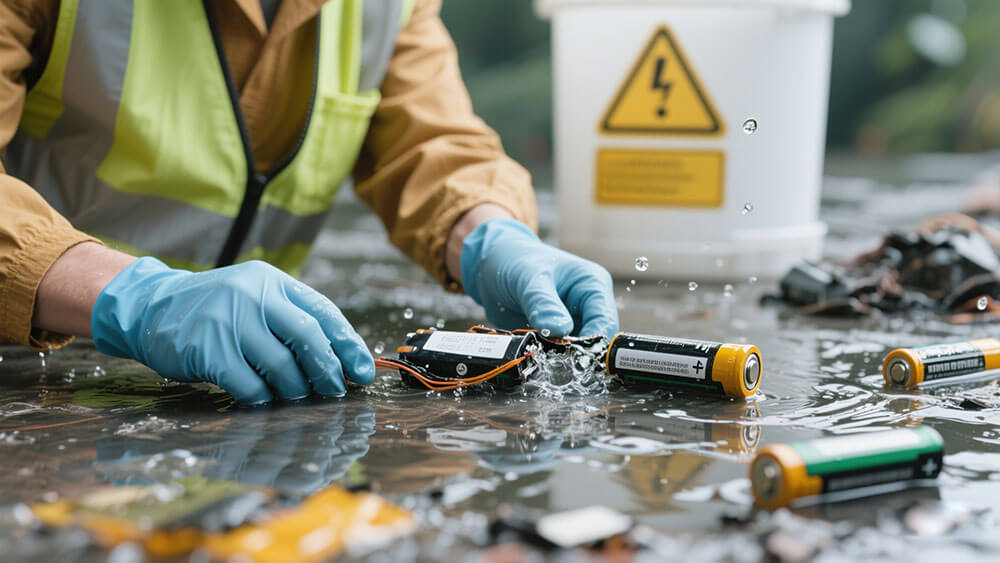
Part 4: Mitigating Environmental Challenges in Battery Recycling
4.1 Role of Renewable Energy in Lithium-Ion Battery Recycling Processes
Integrating renewable energy into battery recycling processes significantly reduces environmental impacts. By powering recycling facilities with solar, wind, or hydroelectric energy, you can lower greenhouse gas (GHG) emissions and energy consumption. For instance:
Recycling operations using renewable energy can reduce GHG emissions to 37.2 kgCO2eq/kWh, a 32% reduction under the SPS scenario.
Under the SDS scenario, emissions drop further to 30.7 kgCO2eq/kWh, representing a 44% reduction.
These reductions highlight the importance of renewable energy in achieving sustainable recycling. Additionally, emissions data from battery production and end-of-life processes confirm the reliability of these findings. By adopting renewable energy, you not only minimize environmental harm but also enhance the overall efficiency of reuse and recycling efforts.
4.2 Supply Chain Optimization for Sustainable Battery Recycling
Optimizing the supply chain is crucial for sustainable battery recycling. A streamlined supply chain reduces transportation emissions, improves material recovery rates, and ensures compliance with environmental standards. You can achieve this by:
Establishing regional recycling hubs to minimize transportation distances.
Implementing digital tracking systems to monitor battery life cycles and recycling progress.
Collaborating with suppliers to standardize battery designs, simplifying disassembly and material recovery.
These strategies enhance the efficiency of reuse and recycling processes while reducing waste. A well-optimized supply chain not only supports environmental goals but also lowers operational costs, making battery recycling more economically viable.
4.3 Policy and Industry Collaboration to Address Environmental Impacts
Strong policies and industry collaboration play a vital role in mitigating the environmental challenges of battery recycling. Governments can enforce regulations that mandate recycling and promote the use of eco-friendly technologies. Meanwhile, industry stakeholders can work together to develop innovative solutions. Key initiatives include:
Incentivizing the adoption of advanced recycling technologies through subsidies or tax benefits.
Establishing public-private partnerships to fund research on sustainable recycling methods.
Creating global standards for battery design and recycling processes to ensure consistency.
By fostering collaboration, you can address the environmental impacts of battery recycling more effectively. These efforts not only benefit the environment but also strengthen the circular economy, ensuring the long-term sustainability of battery technologies.
Lithium-ion battery recycling faces significant environmental challenges, including high energy consumption, toxic waste, and inefficiencies in material recovery. These issues impact industries like electric vehicles, where sustainable practices are critical. For example, only 5-10% of lithium-ion batteries are recycled globally, while over 15 million metric tons of used batteries could accumulate by 2030.
Advancing recycling technologies and integrating renewable energy can mitigate these challenges. Hydrometallurgical recycling reduces CO₂ emissions by up to 70%, offering a sustainable alternative to mining. By adopting innovative methods, you can enhance recycling rates and reduce the environmental footprint of electric vehicles.
Collaboration among industry stakeholders is essential. Governments and businesses must establish global standards, incentivize eco-friendly technologies, and optimize supply chains. Together, these efforts will ensure a sustainable future for electric vehicles and other industries reliant on lithium-ion batteries.
🌱 Tip: Explore custom battery solutions tailored to your industry needs. Learn more from Large Power.
FAQ
What are the main environmental benefits of recycling lithium-ion batteries?
Recycling lithium-ion batteries conserves resources, reduces mining impacts, and minimizes toxic waste. It supports sustainability by recovering valuable materials like lithium and cobalt. Learn more about lithium-ion batteries.
How does battery recycling compare to mining in terms of energy consumption?
Recycling uses significantly less energy than mining. For example, pyrometallurgy consumes 4.59 MJ/kg, while mining virgin materials requires 297.51 MJ/kg. This makes recycling a more sustainable option.
How can Large Power help industries optimize battery recycling?
Large Power offers custom battery solutions tailored to your industry needs. These solutions enhance recycling efficiency and sustainability.