Contents
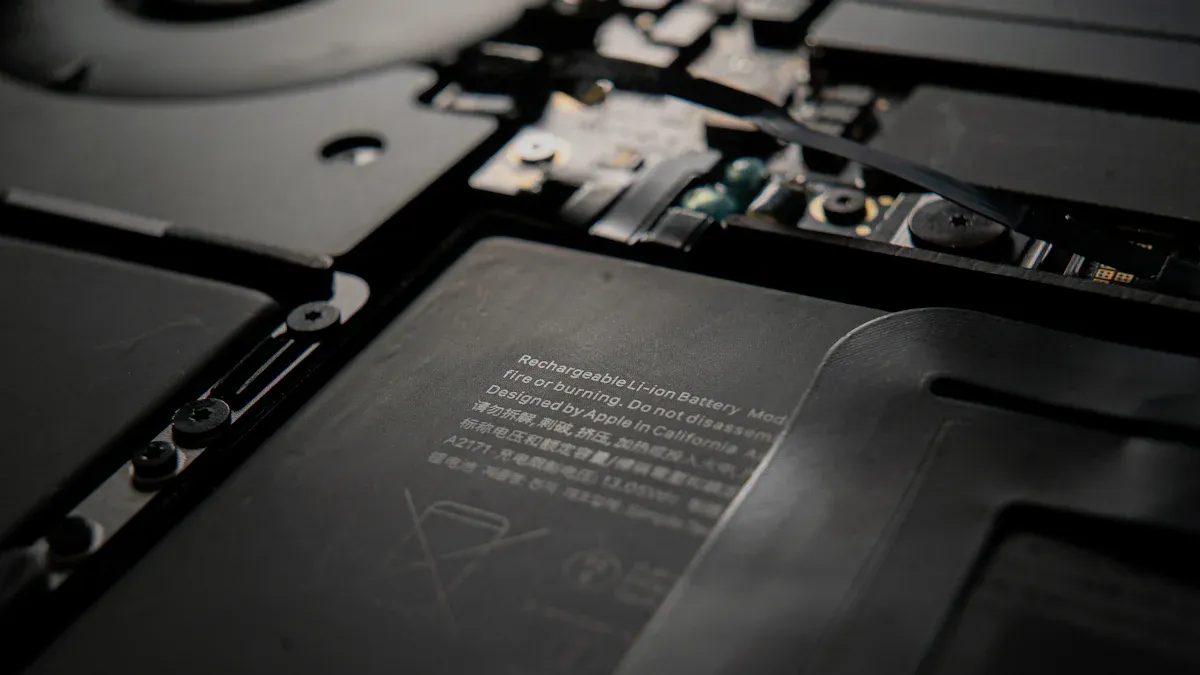
Choosing the correct size of lithium battery is crucial for achieving optimal performance and safety. A properly sized lithium-ion battery ensures efficient energy usage, reduces unnecessary costs, and minimizes risks associated with overloading or underpowering your devices. You need to evaluate several factors, such as your energy consumption, backup requirements, and potential future needs, to make an informed decision. Lithium-ion battery sizing also involves understanding technical specifications like capacity, voltage, and discharge rates. By addressing these aspects, you can maximize the battery’s lifespan and reliability.
Key Takeaways
Figure out how much energy you use daily. List all devices and their power needs to find the total.
Find backup time by dividing battery size by daily energy use. This shows how long the battery lasts during power cuts.
Include energy lost when charging and using the battery. Adjust your math with a factor to match real needs.
Pick the right voltage for your battery setup. Higher voltage lowers energy loss and works better for big systems.
Take care of your battery system often. Use a Battery Management System (BMS) and check for problems to make it last longer and stay safe.
Understanding Load Requirements
Determining the correct size of lithium battery begins with understanding your load requirements. This step ensures that your energy storage system can meet your daily energy load efficiently and reliably. By analyzing energy consumption, backup time, and energy losses, you can calculate the size of the battery pack that fits your needs.
Calculating Energy Consumption
To calculate the size of lithium battery required, you must first identify the application and assess your total daily energy load. Start by listing all devices or systems powered by the battery. For each device, note its power rating (in watts) and the number of hours it operates daily. Multiply these values to find the energy requirement for each device, then sum them up to determine the total daily energy load.
Tip: Use end-use load profiles to refine your calculations. These profiles represent energy consumption patterns across residential and commercial buildings, categorized by building types and climate zones. For example:
Residential profiles simulate single-family homes in various climate regions.
Commercial profiles are based on DOE Commercial Prototype Models.
This data helps you align your calculations with real-world scenarios, ensuring accuracy.
Determining Backup Time
Backup time refers to the duration your battery can supply power during outages or peak demand periods. To calculate this, divide the total battery capacity (in watt-hours) by your daily energy load. For instance, if your battery size is 10,000 watt-hours and your daily energy load is 2,000 watt-hours, your backup time is approximately 5 hours.
Note: Variations in load requirements across different energy usage scenarios can impact backup time. Extensive datasets, such as electric load data from 11 utilities and 2.3 million meters, provide insights into these variations, helping you plan for realistic energy storage needs.
Accounting for Energy Losses
Energy losses occur during charging and discharging processes, reducing the effective amp-hour requirement of your battery. Lithium-ion battery systems typically experience losses due to internal resistance and heat generation. To account for these losses, multiply your calculated energy requirement by a factor (e.g., 1.1 or 1.2) based on the efficiency of your battery system.
Advanced methods, such as machine learning, can improve accuracy in estimating energy losses. By analyzing voltage curves and thermodynamic characteristics, these techniques optimize battery performance and longevity. Incorporating such data-driven approaches ensures your calculations remain reliable over time.
Lithium-Ion Battery Sizing and Capacity
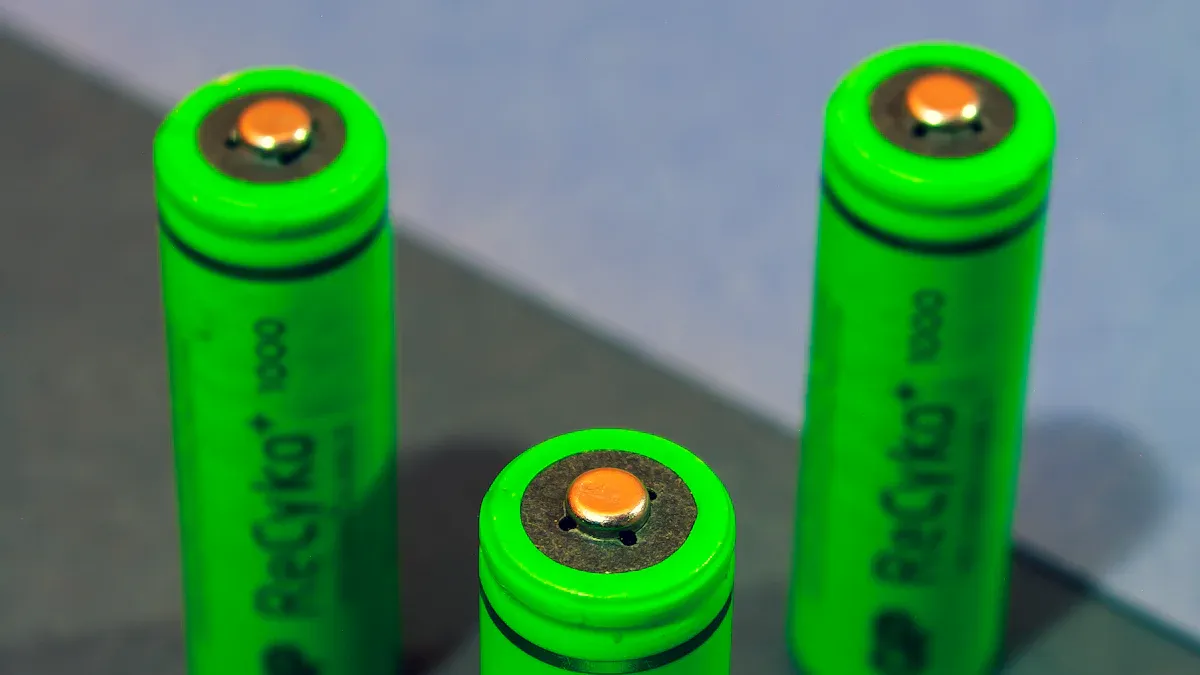
Formula for Calculating Battery Capacity
To determine the size of lithium battery required, you need to calculate its capacity accurately. Battery capacity is typically measured in amp-hours (Ah) or watt-hours (Wh). The formula for calculating total battery capacity is:
Battery Capacity (Ah) = Energy Requirement (Wh) ÷ System Voltage (V)
For example, if your energy requirement is 5,000 Wh and your system voltage is 48V, the battery capacity would be approximately 104 Ah. This calculation ensures your battery pack can meet your energy needs effectively.
Different methods exist for measuring battery capacity, validated by industry standards. The table below highlights these methods:
Method | Description |
---|---|
Charge/Discharge Cycle | Traditional method for measuring capacity, though impractical for large batteries. |
Reserve Capacity (RC) | Reflects runtime at a steady discharge of 25A, as specified by SAE. |
Ah Rating | Indicates capacity at a typical discharge rate, marked by DIN and IEC. |
RC to Ah Conversion | Common formula: RC/2 + 16 or RC/1.9 for estimation. |
Understanding these methods helps you align your calculations with industry benchmarks, ensuring accuracy.
Adjusting for Depth of Discharge (DoD)
Depth of Discharge (DoD) refers to the percentage of a battery’s capacity that has been used. Adjusting for DoD is crucial for extending the lifespan of your lithium battery bank. A lower DoD results in more charge-discharge cycles, enhancing battery longevity. The chart below illustrates how DoD impacts the cycle life of lithium-ion batteries:
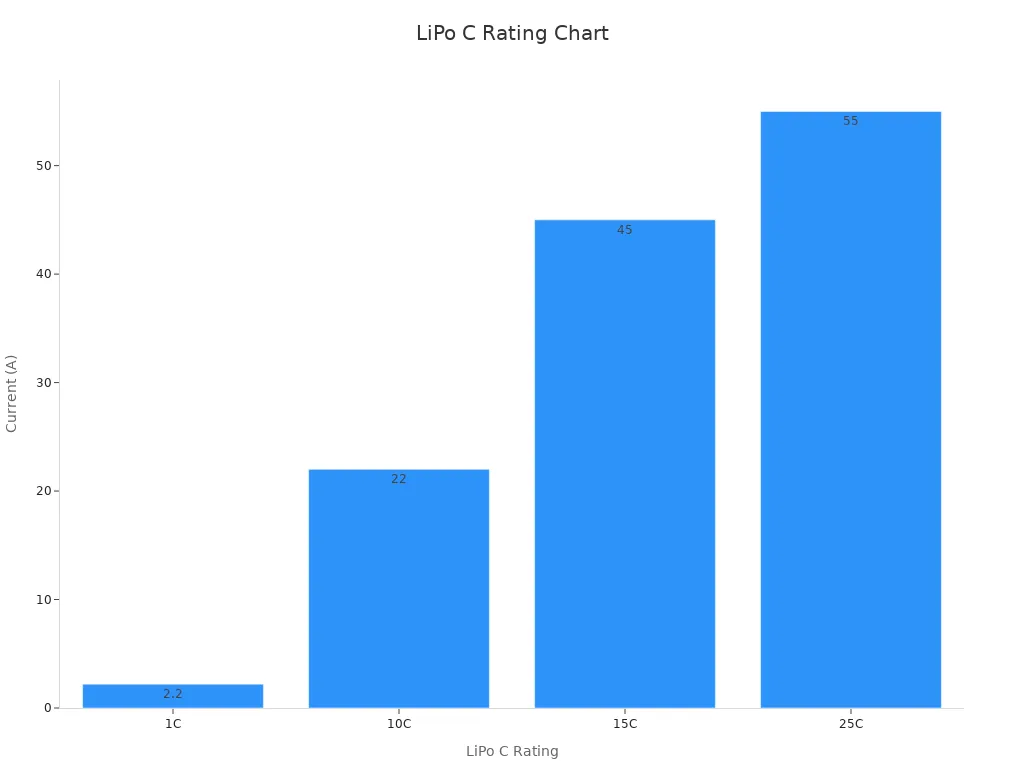
For instance, a battery discharged to 80% DoD may last 900 cycles, while one discharged to 40% DoD could last up to 3,000 cycles. By limiting the DoD, you can optimize the amp-hour requirement and improve the efficiency of your battery pack.
Planning for Future Energy Needs
When sizing a lithium battery bank, consider future energy requirements. Energy consumption trends indicate a growing demand for scalable battery systems. For example:
Global demand for battery cells is projected to reach 2,600 GWh by 2030.
By 2040, this demand could rise to approximately 5,500 GWh under the SSP2 scenario.
Planning for these trends ensures your battery size accommodates potential increases in energy usage. Additionally, advancements in production technology and sustainability practices will play a key role in meeting future energy needs efficiently.
By factoring in these considerations, you can select a lithium battery bank that not only meets your current requirements but also adapts to future demands.
Voltage Selection and Battery Bank Sizing
Choosing the Right Voltage
Selecting the appropriate voltage for your lithium battery bank is essential for optimizing performance and ensuring compatibility with your energy system. Voltage impacts the efficiency, capacity, and lifespan of your battery pack. For most residential and commercial applications, common system voltages include 12V, 24V, and 48V. Higher voltages, such as 48V, are often preferred for larger systems because they reduce current flow, minimizing energy losses and allowing for thinner cables.
Research confirms that voltage behavior during charging and discharging plays a critical role in estimating battery capacity and monitoring health. The thermodynamic and kinetic characteristics of lithium-ion batteries influence these voltage behaviors, making them vital for accurate capacity estimation. Additionally, voltage fluctuations during charge/discharge cycles serve as key indicators of battery degradation, directly affecting the lifespan and overall performance of your battery bank.
Series vs. Parallel Configurations
When designing your battery bank sizing, you must decide between series and parallel configurations. Connecting batteries in series increases the voltage while maintaining the same capacity. For example, connecting four 12V batteries in series results in a 48V system. This setup is ideal for applications requiring higher voltages, such as solar power systems or electric vehicles.
In contrast, parallel configurations increase the capacity while keeping the voltage constant. This approach works well when higher currents are needed. For instance, connecting four 12V batteries in parallel provides the same 12V output but with increased amp-hour capacity. However, a failing cell in a parallel setup can reduce the total load capability, so regular monitoring is crucial.
Combining Series and Parallel Connections
For more complex energy needs, you can combine series and parallel connections. This method allows you to achieve both higher voltage and greater capacity. For example, connecting two sets of four 12V batteries in series (to create two 48V strings) and then connecting these strings in parallel results in a system with both high voltage and increased capacity.
Empirical testing highlights several considerations when combining these configurations. Tapping into a series string can lead to cell imbalance unless corrected by a specialized charger. Parallel connections offer design flexibility but require careful monitoring to avoid safety hazards caused by weak or shorted cells. By understanding these methodologies, you can design a lithium battery bank that meets your specific energy requirements while ensuring safety and efficiency.
Safety Features and Considerations
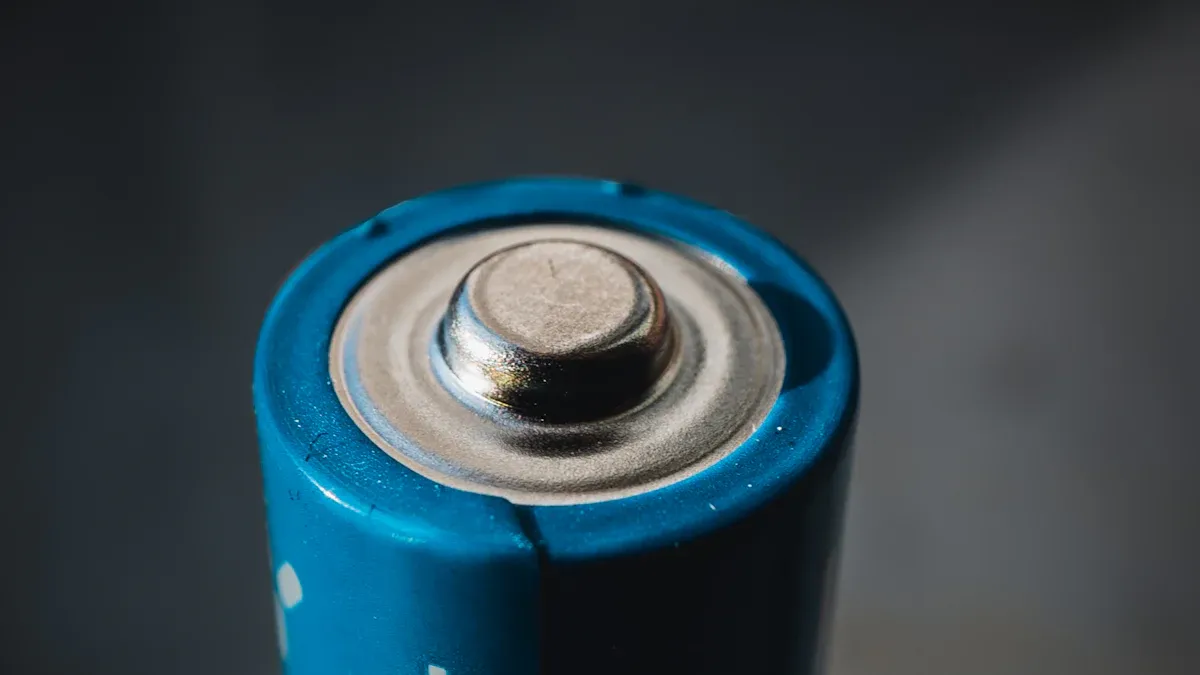
Built-in Safety Mechanisms
Lithium-ion batteries incorporate advanced safety mechanisms to ensure reliable performance and prevent failures. Manufacturers design these batteries with multiple protective layers, including limiting active material, integrating safety features within the cell, and adding electronic protection circuits. These measures significantly reduce the risk of heat-related failures, which are rare in modern lithium-ion batteries.
Key safety mechanisms include:
Fusible Structures: These built-in devices prevent overheating and battery failure.
Thermal Protection: Innovations block ion transport when temperatures rise to dangerous levels.
Redox Shuttles: These molecules absorb excess energy to prevent overcharging.
Additionally, lithium-ion batteries comply with rigorous safety standards such as UL 1642 and IEC 61960, which ensure their reliability across various applications. These certifications validate the effectiveness of built-in safety mechanisms, making lithium battery banks a dependable choice for energy storage.
Proper Installation and Usage
Proper installation and usage practices are critical for maintaining battery safety. Always follow manufacturer guidelines to ensure optimal performance. Lithium-ion batteries include features like PTC devices to inhibit high current surges and safety vents to release gas during pressure increases. These components protect the battery pack from damage caused by improper handling.
Avoid charging batteries at temperatures below 0°C (32°F), as this can lead to lithium plating and compromise safety. Use only branded batteries that meet established safety standards. Non-compliant batteries may lack essential safety features, increasing the risk of failure. Regularly inspect your lithium battery bank for signs of wear or damage to prevent potential hazards.
Environmental Factors
Environmental conditions, such as temperature and humidity, significantly impact lithium-ion battery performance. High temperatures accelerate solid electrolyte interphase (SEI) growth, leading to faster aging. Similarly, lithium plating, which causes rapid degradation, depends on temperature, state of charge (SoC), and charging rate. To extend the lifespan of your battery pack, store it in a cool, dry environment and maintain an appropriate SoC.
By understanding these environmental factors, you can optimize the performance and longevity of your lithium battery bank while ensuring safety.
Tips for Maintaining Lithium-Ion Battery Efficiency
Best Practices for Charging and Discharging
To maximize the efficiency of your lithium battery bank, follow best practices for charging and discharging. Lithium-ion batteries operate with a charging efficiency of 95% to 98%, meaning they retain most of the energy input. This efficiency remains consistent throughout their lifespan, unlike lead-acid batteries, which degrade significantly over time.
Use a Battery Management System (BMS) to regulate charge and discharge cycles. The BMS ensures capacity balancing by redistributing charge between cells, maintaining uniform voltage levels.
Charge your battery at the recommended rate. Most lithium batteries support a maximum charge rate of 1C, which is faster than the typical 0.2C rate for lead-acid batteries.
Avoid deep discharges. Keeping the depth of discharge (DoD) low helps prolong battery life and reduces the amp-hour requirement for your pack.
By adhering to these practices, you can optimize your energy storage system and extend its lifespan.
Regular Maintenance and Monitoring
Regular maintenance and monitoring are essential for ensuring the longevity of your lithium battery bank. Advanced strategies like active balancing and machine learning can significantly enhance performance.
Strategy | Description | Impact on Battery Lifespan |
---|---|---|
Active Balancing | Redistributes charge from higher capacity cells to lower capacity cells. | Enhances battery performance and longevity. |
Machine Learning (ML) | Analyzes historical and environmental data to predict remaining useful life. | Enables proactive maintenance and optimized charging strategies. |
Active balancing ensures that all cells in your battery bank maintain a consistent state of charge, preventing overuse of individual cells. Machine learning tools can predict potential issues, allowing you to address them before they impact performance. Regularly inspect your battery for physical damage or irregularities to avoid unexpected failures.
Proper Storage Conditions
Proper storage conditions play a critical role in maintaining the efficiency of your lithium battery bank. Temperature and state of charge (SoC) are key factors that influence battery performance over time.
Temperature | Lithium-ion (Li-cobalt) at 40% charge | Lithium-ion (Li-cobalt) at 100% charge |
---|---|---|
0°C | 98% | 94% |
25°C | 96% | 80% |
40°C | 85% | 65% |
60°C | 75% | 60% |
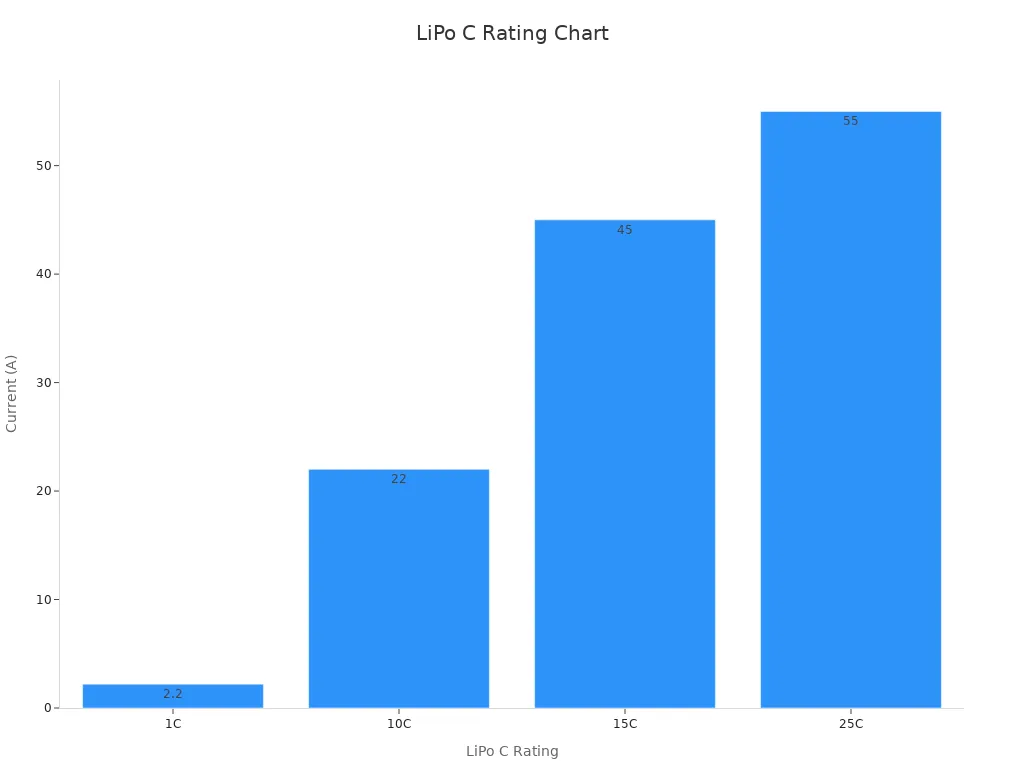
Store your battery in a cool, dry environment to minimize degradation. For long-term storage, maintain a 40% SoC to reduce stress on the cells. Avoid exposing the battery to extreme temperatures, as this accelerates aging and reduces total battery capacity. By following these guidelines, you can prolong battery life and ensure consistent performance.
Choosing the right lithium battery bank involves several critical steps. You must evaluate your load requirements, calculate the appropriate capacity, select the correct voltage, and prioritize safety features. These steps ensure your energy system operates efficiently and reliably. Proper maintenance is equally important to prolong battery life. Regular monitoring, active balancing, and using a battery management system (BMS) can significantly enhance performance and capacity. These practices also help prevent issues like thermal runaway, which can compromise safety.
Professional advisory services provide valuable insights into optimizing your system. Experts can guide you in implementing advanced techniques, such as machine learning, to improve the state of health across your battery pack. This approach not only prolongs battery life but also ensures reliability over time. By following these guidelines, you can maximize the lifespan of your lithium battery bank and avoid unnecessary risks.
Contact Large Power if you have any custom battery pack inquiry regarding your DIY and new product design.
Tip: Regular maintenance and professional advice can prevent costly repairs and extend the life of your battery system.
FAQ
1. How do I know if my lithium-ion battery is the right size for my application?
Evaluate your energy consumption, backup time, and future needs. Use the formula for battery capacity and adjust for depth of discharge (DoD). Ensure compatibility with your system voltage and configuration.
Tip: Consult a professional if you’re unsure about technical specifications or calculations.
2. What happens if I oversize or undersize my battery bank?
Oversizing increases costs unnecessarily, while undersizing risks overloading and reducing efficiency. Both scenarios can shorten battery lifespan and compromise performance.
Note: Proper sizing ensures optimal energy usage and safety.
3. Can I expand my battery bank later if my energy needs grow?
Yes, lithium-ion battery banks can be expanded by adding more batteries in series or parallel. Ensure compatibility with your existing system and monitor for cell imbalances.
4. What is the best way to store lithium-ion batteries long-term?
Store batteries in a cool, dry environment at 40% state of charge (SoC). Avoid extreme temperatures and high humidity to prevent degradation.
5. Are lithium-ion batteries safe for residential use?
Yes, modern lithium-ion batteries include built-in safety mechanisms like thermal protection and electronic circuits. Follow installation guidelines and use certified batteries to ensure safety.
Alert: Regular inspections help identify potential issues early.